Difference between revisions of "Possible consequences of eutrophication"
(→Aesthetic impacts) |
Dronkers J (talk | contribs) |
||
(50 intermediate revisions by 3 users not shown) | |||
Line 1: | Line 1: | ||
+ | |||
+ | |||
==Introduction== | ==Introduction== | ||
− | + | Enhanced plant production and improved fish yields are sometimes described as positive impacts of [[Eutrophication|eutrophication]], especially in countries where fish and other aquatic organisms are a significant source of food<ref>Nixon, S.W. and Buckley, B. A. 2002. “A strikingly rich zone” – Nutrient enrichment and secondary production in coastal marine ecosystems. Estuaries 25: 782–796</ref>. However, detrimental ecological impacts can in turn have other negative consequences and impacts which are described below. The entire aquatic [[ecosystem]] may change with eutrophication. The diagram below summarizes the eutrophication process and its main causes and consequences. | |
− | Enhanced plant production and improved fish yields are sometimes described as positive impacts of [[Eutrophication|eutrophication]], especially in countries where fish and other aquatic organisms are a significant source of food. However detrimental ecological impacts can in turn have other negative consequences and impacts which are described below. | + | |
− | [[ | + | [[File:eutrocon.png|705|center]] |
+ | |||
==Ecological impacts== | ==Ecological impacts== | ||
− | |||
− | |||
− | |||
− | |||
− | |||
− | === | + | ===Increased biomass of phytoplankton resulting in [[algal bloom]]s=== |
− | < | + | [[File:Plentiful_plankton.jpg|250px|thumb|right|<small>Fig. 1. Envisat satellite image of an algal bloom captured with MERIS (Photo Credit: ESA, 2009)</small>]] |
− | + | [[Phytoplankton]] or microalgae are [[photosynthesis|photosynthesizing]] microscopic organisms. They contain chlorophyll and require sunlight in order to live and grow. Most phytoplankton are buoyant and float in the upper part of the ocean where sunlight penetrates the water. In a balanced ecosystem they provide food for a wide range of organisms such as whales, shrimp, snails and jellyfish. | |
− | + | Among the more important groups are the diatoms, cyanobacteria, dinoflagellates and coccolithophores (see: [[Marine Plankton]]). | |
− | + | Phytoplankton species require inorganic [[Nutrient|nutrients]] such as nitrates, phosphates, and sulfur which they convert into proteins, fats and carbohydrates. When too many of these nutrients (by natural or [[Anthropogenic|anthropogenic]] cause) are available in the water, phytoplankton may grow and multiply very fast forming algal blooms (see [[Algal bloom dynamics]]). Algal blooms may occur in freshwater as well as marine environments. Only one or a small number of phytoplankton species are involved and some blooms discolor (green, yellow-brown or red) the water due to their high density of pigmented cells. Blooms in the ocean may cover a large area and are easily visible in satellite images (Fig. 1) – see the article [[Plankton remote sensing]]. Enclosed and semi-enclosed water bodies (e.g., coastal lagoons) are particularly sensitive to increased nutrient loads (especially nitrogen input from agricultural effluents), potentially leading to ecosystem collapse<ref>Ouaissa, S., Gómez-Jakobsen, F., Yebra, L., Ferrera, I., Moreno-Ostos, E., Dolores Belando, M., Ruiz, J.M. and Mercado, J.M. 2023. Phytoplankton dynamics in the Mar Menor, a Mediterranean coastal lagoon strongly impacted by eutrophication. Marine Pollution Bulletin 192, 115074</ref>. | |
+ | |||
+ | ===Dissolved oxygen depletion or hypoxia=== | ||
+ | Oxygen is required for all life forms on the planet. Oxygen is produced by plants during [[photosynthesis]]. At night, animals and plants, as well as aerobic micro-organisms, respire and so consume oxygen which results in a decrease in dissolved oxygen levels. [[Algal bloom]]s may cause strong fluctuations in dissolved oxygen levels. When the algae population is growing at a fast rate, it may block sunlight from reaching other organisms and cause a decrease of dissolved oxygen levels. When algae die, they are decomposed by bacteria which in this process consume oxygen so that the water can become temporarily hypoxic. In this situation, oxygen respiration can be completely replaced by bacterial sulfate respiration, which generates hydrogen sulfide. Hydrogen sulfide is toxic to most of the benthic macrofauna, including functionally important species such as bioturbators. The loss of bioturbation reduces the downward transport of oxygen into the sediment and makes the sediment less cohesive and more susceptible to resuspension. Consequently, water turbidity increases and the growth of oxygen producing phytobenthic communities is reduced. This implies a dramatic change in ecosystem functioning. The buffer capacity against benthic hypoxia is impaired and the system becomes more vulnerable to the development and persistence of hypoxic events<ref>Rabalais, N., Diaz, R., Levin, L., Turner, R., Gilbert, D. and Zhang, J. 2010. Dynamics and distribution of natural and human-caused hypoxia. Biogeosciences 7:585–619</ref>. | ||
+ | |||
+ | ===Dead zones=== | ||
+ | Zones with extreme hypoxia are called [https://en.wikipedia.org/wiki/Dead_zone_(ecology) dead zones]. Upwelling of nutrient-rich waters may produce 'natural' dead zones in some ocean regions where water mass circulation is weak. These so-called 'oxygen minimum zones' occur below the photic zone, between about 200 - 1500 m depth, and are related to microbial mineralization of sinking organic material<ref name=BG>Breitburg, D., Gregoire, M. Isensee, K. (eds.) 2018. The ocean is losing its breath: Declining oxygen in the world’s ocean and coastal waters. IOC-UNESCO, IOC Technical Series, No. 137 40pp. (IOC/2018/TS/137)</ref>. However, most severe dead zones occur as a result of anthropogenic eutrophication of stratified water masses. Stratification is mainly caused by temperature-induced density differences between surface waters and deeper waters in the ocean; stratification in estuaries and coastal waters is mainly due to the salinity-induced density difference between seawater and river water. When an algal bloom in the surface layer decays, the deeper water layers become laden with sinking dead algae that are decomposed by oxygen consuming bacteria. Stratification inhibits turbulent mixing of the oxygen-rich surface layer into the underlying water layer that therefore becomes depleted from oxygen. | ||
+ | |||
+ | In hypoxic areas microbial pathways can quickly dominate the food web, thereby reducing the energy transfer toward higher trophic levels. This leads to an overall decline of biomass, changes in species composition, and loss of habitat and biodiversity<ref>Diaz, R.J. and Rosenberg, R. 2008. Spreading dead zones and consequences for marine ecosystems. Science 321: 926–929</ref>. Under prolonged hypoxia, the total loss of benthic and pelagic fauna can occur. Dead zones have grown dramatically in size and distribution over the last decades. Globally, the observed number of dead zones has increased more than tenfold since the 1960s, and is still tending to grow<ref name=J15>Jessen, C., Bednarz, V.N., Rix, L., Teichberg, M. and Wild, C. 2015. Marine Eutrophication. In: Armon, R., Hänninen, O. (eds) Environmental Indicators. Springer, Dordrecht</ref>. | ||
+ | |||
+ | [[File:OceanHypoxia.jpg|thumb|550px|left|<small>Fig. 2. Areas with hypoxia in coastal seas (red) and in the oceans (blue). From IOC-UNESCO 2018<ref name=BG/> Creative Commons Licence.</small>]] | ||
+ | |||
+ | Fig. 2 shows a map of coastal and ocean regions with frequently occurring hypoxia. The largest and most persistent dead zones are situated in the Baltic Sea, the Black Sea, the Arabian Sea and the Gulf of Mexico. The size of dead zones decreases during severe storms when strong wind-driven turbulent water motion partially neutralizes stratification. These areas can have detrimental effects on commercially important species, thereby affecting local economies and markets that rely on these natural resources. | ||
+ | |||
+ | Demographic growth and economic development are expected to increase the supply of nutrients<ref>Beusen, A.H.W., Doelman, J.C., Van Beek, L.P.H., Van Puijenbroek, P.J.T.M., M. Mogollon, J., Van Grinsven, H.J.M., Stehfest, E., Van Vuuren, D.P. and Bouwman A.F. 2022. Exploring river nitrogen and phosphorus loading and export to global coastal waters in the Shared Socio-economic pathways. Global Environmental Change 72: 102426</ref>. Oxygen depletion in the ocean and coastal waters and climate change will be further exacerbated through increasing ocean surface temperature and stratification<ref>Baroni, I.R., Palastanga, V. and Slomp, C. P. 2020. Enhanced Organic Carbon Burial in Sediments of Oxygen Minimum Zones Upon Ocean Deoxygenation. Front. Mar. Sci. 6: 839</ref><ref>Li, G., Cheng, L., Zhu, J., Trenberth, K.E., Mann, M.E. and Abraham, J.P. 2020. Increasing ocean stratification over the past half-century. Nature Climate Change https://doi.org/10.1038/s41558-020-00918-2</ref>. In turn, ocean oxygen depletion can generate a positive feedback to climate change through increased production of the greenhouse gas NO<sub>2</sub> by microbial denitrification<ref name=BG/>. | ||
+ | <br clear=all> | ||
+ | |||
+ | ===Eutrophication-driven acidification=== | ||
+ | The respiration of bacteria that decompose dead algae not only causes hypoxia due to oxygen consumption, but also produces CO<sub>2</sub> and thus causes an increase in the carbonic acid content of the water. A sharp increase in acidity (pH even below 7) has been observed in highly eutrophic estuaries and lagoons along the east coast of the US, coinciding with hypoxia<ref>Wallace, R.B., Baumann, H., Grear, J.S., Aller, R.C. and Gobler, C.J. 2014. Coastal ocean acidification: The other eutrophication problem. Estuarine, Coastal and Shelf Science 148: 1-13</ref>. Acidification is an annual feature of these estuaries during summer when algae decay and oxygen depletes. Lowest pH levels (undersaturated with respect to aragonite) occur near the bottom, especially in poorly flushed stratified systems. This poses a serious threat to calcifying benthic organisms, for example scallops and other bivalves that spawn in mid-to-late summer. Eutrophication-driven acidification exacerbates [[ocean acidification]] due to uptake of atmospheric CO<sub>2</sub>. | ||
− | === | + | ===Species biodiversity decreases and the dominant biota changes=== |
− | [[ | + | [[File:FishKill.jpg|250px|thumb|right|<small>Fig. 3. Fish kill (menhaden) due to severe hypoxia (Photo credit: Chris Deacutis, IAN Image library )</small>]] |
− | + | Eutrophication leads to changes in the availability of light and certain nutrients to an ecosystem. The growth of phytoplankton causes increased [[Turbidity|turbidity]] or decreased penetration of light into the lower depths of the water column. This shading can inhibit growth of submerged aquatic plants in coastal waters and affect species which are dependent on them (fish, shellfish). This causes shifts in the species composition so that only the more tolerant species survive and new competitive species invade and out-compete original inhabitants. Examples are macroalgae and their massive biomass which inhibits the growth of other aquatic plants and algal blooms that consists of one type of phytoplankton species because other species are outcompeted. | |
− | + | Oxygen depletion, or [[Hypoxia|hypoxia]], being a common consequence of eutrophication, is a cause of fish kills, especially fish that need high levels of dissolved oxygen (Fig. 3). Although many species have adaptations to low oxygen conditions<ref>Altieri, A.H., Johnson, M.D., Swaminathan, S.D., Nelson, H.R. and Gedan, K.B. 2021. Resilience of Tropical Ecosystems to Ocean Deoxygenation. Trends in Ecology and Evolution 36: 227-238</ref>, fish and crustaceans suffer significant mortality already at 2 mg/l O<sub>2</sub> <ref>Vaquer-Sunyer, R. and Duarte, C.M. 2008. Thresholds of hypoxia for marine biodiversity. PNAS 105: 15452–15457</ref>. There is also evidence that hypoxic conditions promote enhanced phosphorus release, which stimulates the growth of cyanobacteria<ref>Funkey, C.P., Conley, D.J., Reuss, N.S., Humborg, C, Jilbert, T. and Slomp, C.P. 2014. Hypoxia Sustains Cyanobacteria Blooms in the Baltic Sea. Environ. Sci. Technol. 48: 2598−2602</ref>. Many cyanobacteria species produce toxins that are lethal to birds and animals. | |
− | |||
− | + | While low levels of nutrient enrichment may stimulate seagrasses through increased photosynthesis and production, high nutrient enrichment generally leads to negative effects (see [[Seagrass meadows]]). Excessive ammonium may be toxic, while nitrate may inhibit growth due to carbon limitation in some seagrass species. Although nutrient stress is not highly detrimental to seagrasses, reduction of light due to algal overgrowth is considered one of the most detrimental indirect effects of eutrophication on seagrasses. The die-off of [[seagrass meadows]] under low oxygen levels is enhanced by positive feedback from oxygen consuming mineralization, but recolonization by germinating seedlings can be rapid<ref>Greve, T.M., Krause-Jensen, D., Rasmussen, M.B. and Christense, P.B. 2005. Means of rapid eelgrass (Zostera marina L.) recolonisation in former dieback areas. Aquatic Botany 82: 143-156</ref>. | |
− | [[ | ||
− | |||
− | ==== | + | ===Green tides=== |
− | < | + | Many observations and experiments provide evidence that the occurrence of so-called 'green tides', consisting of marine [[seaweed]]s or macroalgae, is increased in eutrophicated coastal waters<ref name=SZ>Smetacek, V. and Zingone, A. 2013. Green and golden seaweed tides on the rise. Nature 504, doi:10.1038/nature12860</ref>. These blooms are recognizable by large blades of algae that may wash up onto the shoreline (Fig. 4). |
+ | [[File: GreenAlgae_D_Ramirez.jpg|thumb|350px|right|<small>Fig. 4. Macroalgae washed ashore. Photo credit D. Ramirez</small>.]] | ||
+ | Benthic green macroalgae of the genus ''Ulva'' are by far the most commonly cited bloom-forming species under eutrophic conditions worldwide. The isotope <math>δ^{15}N</math> signature of faster growing species, such as ''Ulva sp.'', can indicate changes in their environment or nutrient supply relatively quickly, in a matter of days, while slower growing species such as ''Fucus sp.'' are better long-term indicators of nutrient supply<ref>Teichberg, M., Fox, S.E., Aguila, C., Olsen, Y.S. and Valiela, I, 2008. Macroalgal responses to experimental nutrient enrichment in shallow coastal waters: growth, internal nutrient pools, and isotopic signatures. Mar. Ecol. Prog. Ser. 368: 117–126</ref>. As compared to perennial species, [[seaweed]]s fix carbon in excess and subsequently release large amounts of dissolved organic matter into surrounding water. This organic matter quickly enters the microbial food web, thereby stimulating microbial activity, increasing the biological oxygen demand of bottom waters, and causing hypoxic conditions to occur. Hypoxia and changes in the composition of primary producer species are in turn responsible for the major fauna community shifts that accompany macroalgal blooms in eutrophic areas<ref name=J15/>. They can be particularly harmful to seagrass and tropical coral reef ecosystems as they are highly adapted to [[oligotrophic]] waters, support a high biodiversity, and are important nurseries for many juvenile fish species. Green tides can asphyxiate aquacultures or disrupt traditional artisanal fisheries. Macroalgae can also be valorized as a raw material; harvesting could therefore be an effective solution<ref name=SZ/> (see also [[Seaweed (macro-algae) ecosystem services]]). | ||
+ | <br clear=all> | ||
− | + | ===Increased blooms of gelatinous zooplankton=== | |
− | [[ | + | [[Phytoplankton]] are the food source for numerous other organisms, especially the zooplankton. [[Zooplankton]] are heterotrophic plankton. They are primarily transported by ambient water currents but many can swim. Through their consumption and processing of phytoplankton and other food sources they play a role in aquatic food webs as a resource for higher trophic levels including fish. Zooplankton can be divided in two important groups: crustacean (copepods and krill) and '''gelatinous zooplankton'''. Gelatinous zooplankton have relatively fragile, plastic gelatinous bodies that contain at least 95% water and which lack rigid skeletal parts. The most well-known are the jellyfish. There is some evidence that gelatinous zooplankton organisms increase in relative importance versus crustacean zooplankton in areas where the natural species diversity has been affected by eutrophocation, over-fishing and climate change<ref> Condon, R.H., Duarte, C.M., Pitt, K.A., Robinson, K.L., Lucas, C.H., Sutherland, K.R., Mianzan, H.W., Bogeberg, M., Purcell, J.E., Decker, M.B., Uye, S.-I., Madin, L.P., Brodeur, R.D., Haddock, S.H.D., Malej, A., Parry, G.D., Eriksen, E., Quiñones, J., Acha, M., Harvey, M., Arthur, J.M. and Graham W.M. 2013. Recurrent jellyfish blooms are a consequence of global oscillations. PNAS 110: 1000-1005</ref>. |
− | + | ||
− | + | ===Toxic or inedible phytoplankton species ([[harmful algal blooms]])=== | |
+ | '''Harmful algal blooms (HAB)''' are bloom events involving '''toxic or harmful phytoplankton'''. These cause harm through the production of toxins or by their accumulated biomass, which can effect co-occurring organisms and alter food web dynamics. Impacts include: | ||
+ | *Human illness, | ||
+ | *Mortality of fish, birds and mammals following consumption or indirect exposure to HAB toxins, | ||
+ | *Substantially economic losses to coastal communities and commercial fisheries. | ||
+ | For more details on toxic algal blooms, see the article [[Harmful algal bloom]]. | ||
==Human health impacts== | ==Human health impacts== | ||
− | + | Harmful algal bloom species have the capacity to produce '''toxins''' dangerous to humans. Algal [[Toxic|toxins]] are observed in marine ecosystems where they can accumulate in shellfish and more generally in seafood reaching dangerous levels for human as well as animal health. Examples include paralytic, neurotoxic and diarrhoeic shellfish poisoning. Several algal species able of producing toxins harmful to human or marine life have been identified in European coastal waters<ref>Eutrophication and health. European Commission 2002. Office for Official Publications of the European Communities: Luxembourg. ISBN 92-894-4413-4.28 pp.</ref>. The table gives an overview of some species that are regularly observed and represent a risk for seafood consumers<ref name=B16>Berdalet, E., Fleming, L.E., Gowen, R., Davidson, K., Hess, P., Backer, L.C., Moore, S.K., Hoagland, P. and Enevoldsen, H. 2016. Marine harmful algal blooms, human health and wellbeing: challenges and opportunities in the 21st century. J. Mar. Biol. Assoc. U.K. 96: 61–91</ref>. | |
+ | |||
+ | |||
{|border="1" align=center cellspacing="0" cellpadding = "8" width="825px" | {|border="1" align=center cellspacing="0" cellpadding = "8" width="825px" | ||
− | !style="background-color:# | + | !style="background-color:#EEEEAA" |'''Disease''' |
− | !style="background-color:# | + | !style="background-color:#EEEEAA" |'''Symptoms''' |
− | !style="background-color:# | + | !style="background-color:#EEEEAA" |'''Species''' |
− | !style="background-color:# | + | !style="background-color:#EEEEAA" |'''Carriers''' |
|- | |- | ||
| '''A'''mnesic '''s'''hellfish '''p'''oisoning (ASP) | | '''A'''mnesic '''s'''hellfish '''p'''oisoning (ASP) | ||
Line 70: | Line 93: | ||
|- | |- | ||
|} | |} | ||
− | + | ||
+ | |||
+ | Finfish can also be a vector for toxins, for example [https://en.wikipedia.org/wiki/Ciguatera_fish_poisoning ciguatoxins], where it is typically predator fish whose flesh is contaminated with the toxins originally produced by dinoflagellates<ref name=B16/>. People are poisoned when consuming contaminated fish. Symptoms include gastrointestinal and neurological effects. | ||
==Socio-economic impacts== | ==Socio-economic impacts== | ||
Nearly all of the above described impacts have a direct or indirect socio-economic impact. | Nearly all of the above described impacts have a direct or indirect socio-economic impact. | ||
− | ==== Aesthetic impacts=== | + | |
+ | ===Impact on recreation and tourism=== | ||
+ | The enrichment of nutrients to an ecosystem can result in a massive growth of macroalgae. The existence of such dense algal growth areas can inhibit or prevent access to waterways. The seaweed is harmless to humans but decreases the fitness for use of the water for water sports (swimming, boating and fishing). | ||
+ | |||
+ | ===Aesthetic impacts=== | ||
+ | [[Image:Beach_closed.jpg|200px|thumb|right|<small>Fig. 5. Beaches can be closed as a result of toxic algal blooms (Photo credit: Elizabeth Halliday, Woods Hole Oceanographic Institution).</small>]] | ||
Algal blooms are unsightly and can have unpleasant smells for example: | Algal blooms are unsightly and can have unpleasant smells for example: | ||
− | * The appearance of a | + | * The appearance of a white yellowish foam on the beach in spring, for example on the shores along the North Sea. The foam is formed by the wind that sweeps up the decaying remains of ''Phaeocystis'' algal colonies. An extreme case is shown in [[Foam beach, Sydney]]. |
− | * When macroalgae or seaweed are decomposed by anaerobic bacteria hydrogen sulfide | + | * When macroalgae or seaweed are decomposed by anaerobic bacteria hydrogen, sulfide (H<sub>2</sub>S) is released. This gas is characterized by a very unpleasant characteristic foul odor of rotten eggs. |
+ | |||
+ | ===Economic impacts=== | ||
+ | Due to the toxins produced by harmful algal blooms commercial fish and shellfish may become '''unsuitable for consumption''' resulting in potential economic and financial problems for the fishing industries. In extreme cases beaches are closed due to the presence of toxic algal blooms (Fig. 5). The annual economic costs related to illness and death caused by consumption of poisoned fish products is estimated at around 30 million US$ worldwide<ref>Kouakou, C.R.C. and Poder, T.G. 2019. Economic impact of harmful algal blooms on human health: a systematic review. Journal of Water and Health 17.4: 499-516</ref>. The fishing industry is suffering economic losses because consumers refrain from consuming fish and shellfish products due to the perceived danger of poisoning. For the USA, the loss related to this avoidance behavior is estimated at around 1 billion US$ per year<ref>Parsons, G.R., Morgan, A.O., Whitehead, J.C. and Haab, T.C. 2006. The Welfare Effects of Pfiesteria-Related Fish Kills: A Contingent Behavior Analysis of Seafood Consumers. Agricultural and Resource Economics Review 35(1)</ref>. | ||
+ | |||
+ | The Baltic Sea harbours one of the the largest dead zones in the world. Lost revenues from ecosystem services (fishing, recreation, but also non-use values) as a result of eutrophication amount to 3-4 billion euros, according to an estimate by HELCOM<ref>Hyytiainen, K., Hasler, B., Ericsdotter, S., Nekoro, M., Blyh, K., Artell, J., Ahlvik, L. and Ahtiainen, J. 2013. Worth it: Benefits outweigh costs in reducing eutrophication in the Baltic. BalticSTERN Summary Report for HELCOM 2013 Ministerial Meeting</ref>. | ||
+ | |||
+ | Another consequence of eutrophication is enhanced biofouling, which accelerates the degradation of marine equipment and infrastructures<ref>Guo, D., Wang, Y., Zhang, Y., Duan, J., Guan, F. and Hou, B. 2024. Effects of marine eutrophication environment on microbial corrosion: A review. Marine Pollution Bulletin 205, 116637</ref>. This leads to additional costs for the marine industry and promotes the use of environmentally unfriendly antifouling agents. | ||
+ | |||
+ | |||
+ | |||
+ | ==Related articles== | ||
+ | :[[Harmful algal bloom]] | ||
+ | :[[Nutrient conversion in the marine environment]] | ||
+ | :[[Algal bloom dynamics]] | ||
+ | :[[What causes eutrophication?]] | ||
+ | :[[Threats to the coastal zone]] | ||
+ | :[[Estuarine turbidity maximum]] | ||
+ | :[[Case studies eutrophication]] | ||
+ | :[[Coupled hydrodynamic - water quality - ecological modelling]] | ||
− | [[ | + | See also other articles in the [[:Category:Eutrophication]] |
− | |||
− | |||
− | |||
− | |||
− | |||
− | == References== | + | ==References== |
− | + | <references/> | |
− | |||
− | |||
− | |||
− | |||
− | |||
− | |||
− | |||
{{author | {{author | ||
|AuthorID=26102 | |AuthorID=26102 | ||
|AuthorFullName= Knockaert, Carolien | |AuthorFullName= Knockaert, Carolien | ||
|AuthorName=Carolienk}} | |AuthorName=Carolienk}} | ||
+ | |||
+ | |||
+ | {{Review | ||
+ | |name=Job Dronkers|AuthorID=120| | ||
+ | }} | ||
+ | |||
+ | [[Category:Eutrophication]] |
Latest revision as of 17:59, 11 November 2024
Contents
- 1 Introduction
- 2 Ecological impacts
- 2.1 Increased biomass of phytoplankton resulting in algal blooms
- 2.2 Dissolved oxygen depletion or hypoxia
- 2.3 Dead zones
- 2.4 Eutrophication-driven acidification
- 2.5 Species biodiversity decreases and the dominant biota changes
- 2.6 Green tides
- 2.7 Increased blooms of gelatinous zooplankton
- 2.8 Toxic or inedible phytoplankton species (harmful algal blooms)
- 3 Human health impacts
- 4 Socio-economic impacts
- 5 Related articles
- 6 References
Introduction
Enhanced plant production and improved fish yields are sometimes described as positive impacts of eutrophication, especially in countries where fish and other aquatic organisms are a significant source of food[1]. However, detrimental ecological impacts can in turn have other negative consequences and impacts which are described below. The entire aquatic ecosystem may change with eutrophication. The diagram below summarizes the eutrophication process and its main causes and consequences.
Ecological impacts
Increased biomass of phytoplankton resulting in algal blooms
Phytoplankton or microalgae are photosynthesizing microscopic organisms. They contain chlorophyll and require sunlight in order to live and grow. Most phytoplankton are buoyant and float in the upper part of the ocean where sunlight penetrates the water. In a balanced ecosystem they provide food for a wide range of organisms such as whales, shrimp, snails and jellyfish. Among the more important groups are the diatoms, cyanobacteria, dinoflagellates and coccolithophores (see: Marine Plankton). Phytoplankton species require inorganic nutrients such as nitrates, phosphates, and sulfur which they convert into proteins, fats and carbohydrates. When too many of these nutrients (by natural or anthropogenic cause) are available in the water, phytoplankton may grow and multiply very fast forming algal blooms (see Algal bloom dynamics). Algal blooms may occur in freshwater as well as marine environments. Only one or a small number of phytoplankton species are involved and some blooms discolor (green, yellow-brown or red) the water due to their high density of pigmented cells. Blooms in the ocean may cover a large area and are easily visible in satellite images (Fig. 1) – see the article Plankton remote sensing. Enclosed and semi-enclosed water bodies (e.g., coastal lagoons) are particularly sensitive to increased nutrient loads (especially nitrogen input from agricultural effluents), potentially leading to ecosystem collapse[2].
Dissolved oxygen depletion or hypoxia
Oxygen is required for all life forms on the planet. Oxygen is produced by plants during photosynthesis. At night, animals and plants, as well as aerobic micro-organisms, respire and so consume oxygen which results in a decrease in dissolved oxygen levels. Algal blooms may cause strong fluctuations in dissolved oxygen levels. When the algae population is growing at a fast rate, it may block sunlight from reaching other organisms and cause a decrease of dissolved oxygen levels. When algae die, they are decomposed by bacteria which in this process consume oxygen so that the water can become temporarily hypoxic. In this situation, oxygen respiration can be completely replaced by bacterial sulfate respiration, which generates hydrogen sulfide. Hydrogen sulfide is toxic to most of the benthic macrofauna, including functionally important species such as bioturbators. The loss of bioturbation reduces the downward transport of oxygen into the sediment and makes the sediment less cohesive and more susceptible to resuspension. Consequently, water turbidity increases and the growth of oxygen producing phytobenthic communities is reduced. This implies a dramatic change in ecosystem functioning. The buffer capacity against benthic hypoxia is impaired and the system becomes more vulnerable to the development and persistence of hypoxic events[3].
Dead zones
Zones with extreme hypoxia are called dead zones. Upwelling of nutrient-rich waters may produce 'natural' dead zones in some ocean regions where water mass circulation is weak. These so-called 'oxygen minimum zones' occur below the photic zone, between about 200 - 1500 m depth, and are related to microbial mineralization of sinking organic material[4]. However, most severe dead zones occur as a result of anthropogenic eutrophication of stratified water masses. Stratification is mainly caused by temperature-induced density differences between surface waters and deeper waters in the ocean; stratification in estuaries and coastal waters is mainly due to the salinity-induced density difference between seawater and river water. When an algal bloom in the surface layer decays, the deeper water layers become laden with sinking dead algae that are decomposed by oxygen consuming bacteria. Stratification inhibits turbulent mixing of the oxygen-rich surface layer into the underlying water layer that therefore becomes depleted from oxygen.
In hypoxic areas microbial pathways can quickly dominate the food web, thereby reducing the energy transfer toward higher trophic levels. This leads to an overall decline of biomass, changes in species composition, and loss of habitat and biodiversity[5]. Under prolonged hypoxia, the total loss of benthic and pelagic fauna can occur. Dead zones have grown dramatically in size and distribution over the last decades. Globally, the observed number of dead zones has increased more than tenfold since the 1960s, and is still tending to grow[6].
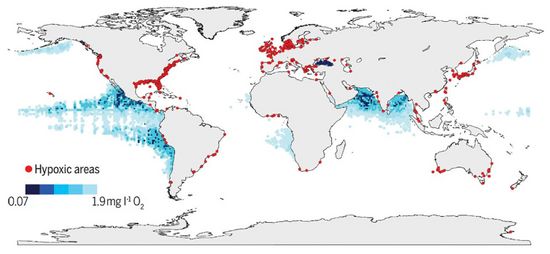
Fig. 2 shows a map of coastal and ocean regions with frequently occurring hypoxia. The largest and most persistent dead zones are situated in the Baltic Sea, the Black Sea, the Arabian Sea and the Gulf of Mexico. The size of dead zones decreases during severe storms when strong wind-driven turbulent water motion partially neutralizes stratification. These areas can have detrimental effects on commercially important species, thereby affecting local economies and markets that rely on these natural resources.
Demographic growth and economic development are expected to increase the supply of nutrients[7]. Oxygen depletion in the ocean and coastal waters and climate change will be further exacerbated through increasing ocean surface temperature and stratification[8][9]. In turn, ocean oxygen depletion can generate a positive feedback to climate change through increased production of the greenhouse gas NO2 by microbial denitrification[4].
Eutrophication-driven acidification
The respiration of bacteria that decompose dead algae not only causes hypoxia due to oxygen consumption, but also produces CO2 and thus causes an increase in the carbonic acid content of the water. A sharp increase in acidity (pH even below 7) has been observed in highly eutrophic estuaries and lagoons along the east coast of the US, coinciding with hypoxia[10]. Acidification is an annual feature of these estuaries during summer when algae decay and oxygen depletes. Lowest pH levels (undersaturated with respect to aragonite) occur near the bottom, especially in poorly flushed stratified systems. This poses a serious threat to calcifying benthic organisms, for example scallops and other bivalves that spawn in mid-to-late summer. Eutrophication-driven acidification exacerbates ocean acidification due to uptake of atmospheric CO2.
Species biodiversity decreases and the dominant biota changes
Eutrophication leads to changes in the availability of light and certain nutrients to an ecosystem. The growth of phytoplankton causes increased turbidity or decreased penetration of light into the lower depths of the water column. This shading can inhibit growth of submerged aquatic plants in coastal waters and affect species which are dependent on them (fish, shellfish). This causes shifts in the species composition so that only the more tolerant species survive and new competitive species invade and out-compete original inhabitants. Examples are macroalgae and their massive biomass which inhibits the growth of other aquatic plants and algal blooms that consists of one type of phytoplankton species because other species are outcompeted.
Oxygen depletion, or hypoxia, being a common consequence of eutrophication, is a cause of fish kills, especially fish that need high levels of dissolved oxygen (Fig. 3). Although many species have adaptations to low oxygen conditions[11], fish and crustaceans suffer significant mortality already at 2 mg/l O2 [12]. There is also evidence that hypoxic conditions promote enhanced phosphorus release, which stimulates the growth of cyanobacteria[13]. Many cyanobacteria species produce toxins that are lethal to birds and animals.
While low levels of nutrient enrichment may stimulate seagrasses through increased photosynthesis and production, high nutrient enrichment generally leads to negative effects (see Seagrass meadows). Excessive ammonium may be toxic, while nitrate may inhibit growth due to carbon limitation in some seagrass species. Although nutrient stress is not highly detrimental to seagrasses, reduction of light due to algal overgrowth is considered one of the most detrimental indirect effects of eutrophication on seagrasses. The die-off of seagrass meadows under low oxygen levels is enhanced by positive feedback from oxygen consuming mineralization, but recolonization by germinating seedlings can be rapid[14].
Green tides
Many observations and experiments provide evidence that the occurrence of so-called 'green tides', consisting of marine seaweeds or macroalgae, is increased in eutrophicated coastal waters[15]. These blooms are recognizable by large blades of algae that may wash up onto the shoreline (Fig. 4).
Benthic green macroalgae of the genus Ulva are by far the most commonly cited bloom-forming species under eutrophic conditions worldwide. The isotope [math]δ^{15}N[/math] signature of faster growing species, such as Ulva sp., can indicate changes in their environment or nutrient supply relatively quickly, in a matter of days, while slower growing species such as Fucus sp. are better long-term indicators of nutrient supply[16]. As compared to perennial species, seaweeds fix carbon in excess and subsequently release large amounts of dissolved organic matter into surrounding water. This organic matter quickly enters the microbial food web, thereby stimulating microbial activity, increasing the biological oxygen demand of bottom waters, and causing hypoxic conditions to occur. Hypoxia and changes in the composition of primary producer species are in turn responsible for the major fauna community shifts that accompany macroalgal blooms in eutrophic areas[6]. They can be particularly harmful to seagrass and tropical coral reef ecosystems as they are highly adapted to oligotrophic waters, support a high biodiversity, and are important nurseries for many juvenile fish species. Green tides can asphyxiate aquacultures or disrupt traditional artisanal fisheries. Macroalgae can also be valorized as a raw material; harvesting could therefore be an effective solution[15] (see also Seaweed (macro-algae) ecosystem services).
Increased blooms of gelatinous zooplankton
Phytoplankton are the food source for numerous other organisms, especially the zooplankton. Zooplankton are heterotrophic plankton. They are primarily transported by ambient water currents but many can swim. Through their consumption and processing of phytoplankton and other food sources they play a role in aquatic food webs as a resource for higher trophic levels including fish. Zooplankton can be divided in two important groups: crustacean (copepods and krill) and gelatinous zooplankton. Gelatinous zooplankton have relatively fragile, plastic gelatinous bodies that contain at least 95% water and which lack rigid skeletal parts. The most well-known are the jellyfish. There is some evidence that gelatinous zooplankton organisms increase in relative importance versus crustacean zooplankton in areas where the natural species diversity has been affected by eutrophocation, over-fishing and climate change[17].
Toxic or inedible phytoplankton species (harmful algal blooms)
Harmful algal blooms (HAB) are bloom events involving toxic or harmful phytoplankton. These cause harm through the production of toxins or by their accumulated biomass, which can effect co-occurring organisms and alter food web dynamics. Impacts include:
- Human illness,
- Mortality of fish, birds and mammals following consumption or indirect exposure to HAB toxins,
- Substantially economic losses to coastal communities and commercial fisheries.
For more details on toxic algal blooms, see the article Harmful algal bloom.
Human health impacts
Harmful algal bloom species have the capacity to produce toxins dangerous to humans. Algal toxins are observed in marine ecosystems where they can accumulate in shellfish and more generally in seafood reaching dangerous levels for human as well as animal health. Examples include paralytic, neurotoxic and diarrhoeic shellfish poisoning. Several algal species able of producing toxins harmful to human or marine life have been identified in European coastal waters[18]. The table gives an overview of some species that are regularly observed and represent a risk for seafood consumers[19].
Disease | Symptoms | Species | Carriers |
---|---|---|---|
Amnesic shellfish poisoning (ASP) | Mental confusion and memory loss, disorientation and sometimes coma | Diatoms of the genus Nitzschia | Shellfish (mussels) |
Neurotoxic shellfish poisoning (NSP) | Muscular paralysis, state of shock and sometimes death | Genus Gymnodinium | Oysters, clams and crustaceans |
Venerupin shellfish poisoning (VSP) | Gastrointestinal, nervous and hemorrhagic, hepatic symptoms and in extreme causes delirium and hepatic coma | Genus Prorocentrum | Oysters and clams |
Diarrhoeic shellfish poisoning (DSP) | Gastrointestinal symptoms (diarrhoea, vomiting and abdominal pain) | Genus Dinophysis and Prorocentrum | Filtering shellfish (oysters, mussels and clams) |
Paralytic shellfish poisoning (PSP) | Muscular paralysis, difficulty in breathing, shock and in extreme causes death by respiratory arrest | Genus Alexandrium and Gymnodinium | Oysters, mussels, crustacean and fish |
Finfish can also be a vector for toxins, for example ciguatoxins, where it is typically predator fish whose flesh is contaminated with the toxins originally produced by dinoflagellates[19]. People are poisoned when consuming contaminated fish. Symptoms include gastrointestinal and neurological effects.
Socio-economic impacts
Nearly all of the above described impacts have a direct or indirect socio-economic impact.
Impact on recreation and tourism
The enrichment of nutrients to an ecosystem can result in a massive growth of macroalgae. The existence of such dense algal growth areas can inhibit or prevent access to waterways. The seaweed is harmless to humans but decreases the fitness for use of the water for water sports (swimming, boating and fishing).
Aesthetic impacts
Algal blooms are unsightly and can have unpleasant smells for example:
- The appearance of a white yellowish foam on the beach in spring, for example on the shores along the North Sea. The foam is formed by the wind that sweeps up the decaying remains of Phaeocystis algal colonies. An extreme case is shown in Foam beach, Sydney.
- When macroalgae or seaweed are decomposed by anaerobic bacteria hydrogen, sulfide (H2S) is released. This gas is characterized by a very unpleasant characteristic foul odor of rotten eggs.
Economic impacts
Due to the toxins produced by harmful algal blooms commercial fish and shellfish may become unsuitable for consumption resulting in potential economic and financial problems for the fishing industries. In extreme cases beaches are closed due to the presence of toxic algal blooms (Fig. 5). The annual economic costs related to illness and death caused by consumption of poisoned fish products is estimated at around 30 million US$ worldwide[20]. The fishing industry is suffering economic losses because consumers refrain from consuming fish and shellfish products due to the perceived danger of poisoning. For the USA, the loss related to this avoidance behavior is estimated at around 1 billion US$ per year[21].
The Baltic Sea harbours one of the the largest dead zones in the world. Lost revenues from ecosystem services (fishing, recreation, but also non-use values) as a result of eutrophication amount to 3-4 billion euros, according to an estimate by HELCOM[22].
Another consequence of eutrophication is enhanced biofouling, which accelerates the degradation of marine equipment and infrastructures[23]. This leads to additional costs for the marine industry and promotes the use of environmentally unfriendly antifouling agents.
Related articles
- Harmful algal bloom
- Nutrient conversion in the marine environment
- Algal bloom dynamics
- What causes eutrophication?
- Threats to the coastal zone
- Estuarine turbidity maximum
- Case studies eutrophication
- Coupled hydrodynamic - water quality - ecological modelling
See also other articles in the Category:Eutrophication
References
- ↑ Nixon, S.W. and Buckley, B. A. 2002. “A strikingly rich zone” – Nutrient enrichment and secondary production in coastal marine ecosystems. Estuaries 25: 782–796
- ↑ Ouaissa, S., Gómez-Jakobsen, F., Yebra, L., Ferrera, I., Moreno-Ostos, E., Dolores Belando, M., Ruiz, J.M. and Mercado, J.M. 2023. Phytoplankton dynamics in the Mar Menor, a Mediterranean coastal lagoon strongly impacted by eutrophication. Marine Pollution Bulletin 192, 115074
- ↑ Rabalais, N., Diaz, R., Levin, L., Turner, R., Gilbert, D. and Zhang, J. 2010. Dynamics and distribution of natural and human-caused hypoxia. Biogeosciences 7:585–619
- ↑ 4.0 4.1 4.2 Breitburg, D., Gregoire, M. Isensee, K. (eds.) 2018. The ocean is losing its breath: Declining oxygen in the world’s ocean and coastal waters. IOC-UNESCO, IOC Technical Series, No. 137 40pp. (IOC/2018/TS/137)
- ↑ Diaz, R.J. and Rosenberg, R. 2008. Spreading dead zones and consequences for marine ecosystems. Science 321: 926–929
- ↑ 6.0 6.1 Jessen, C., Bednarz, V.N., Rix, L., Teichberg, M. and Wild, C. 2015. Marine Eutrophication. In: Armon, R., Hänninen, O. (eds) Environmental Indicators. Springer, Dordrecht
- ↑ Beusen, A.H.W., Doelman, J.C., Van Beek, L.P.H., Van Puijenbroek, P.J.T.M., M. Mogollon, J., Van Grinsven, H.J.M., Stehfest, E., Van Vuuren, D.P. and Bouwman A.F. 2022. Exploring river nitrogen and phosphorus loading and export to global coastal waters in the Shared Socio-economic pathways. Global Environmental Change 72: 102426
- ↑ Baroni, I.R., Palastanga, V. and Slomp, C. P. 2020. Enhanced Organic Carbon Burial in Sediments of Oxygen Minimum Zones Upon Ocean Deoxygenation. Front. Mar. Sci. 6: 839
- ↑ Li, G., Cheng, L., Zhu, J., Trenberth, K.E., Mann, M.E. and Abraham, J.P. 2020. Increasing ocean stratification over the past half-century. Nature Climate Change https://doi.org/10.1038/s41558-020-00918-2
- ↑ Wallace, R.B., Baumann, H., Grear, J.S., Aller, R.C. and Gobler, C.J. 2014. Coastal ocean acidification: The other eutrophication problem. Estuarine, Coastal and Shelf Science 148: 1-13
- ↑ Altieri, A.H., Johnson, M.D., Swaminathan, S.D., Nelson, H.R. and Gedan, K.B. 2021. Resilience of Tropical Ecosystems to Ocean Deoxygenation. Trends in Ecology and Evolution 36: 227-238
- ↑ Vaquer-Sunyer, R. and Duarte, C.M. 2008. Thresholds of hypoxia for marine biodiversity. PNAS 105: 15452–15457
- ↑ Funkey, C.P., Conley, D.J., Reuss, N.S., Humborg, C, Jilbert, T. and Slomp, C.P. 2014. Hypoxia Sustains Cyanobacteria Blooms in the Baltic Sea. Environ. Sci. Technol. 48: 2598−2602
- ↑ Greve, T.M., Krause-Jensen, D., Rasmussen, M.B. and Christense, P.B. 2005. Means of rapid eelgrass (Zostera marina L.) recolonisation in former dieback areas. Aquatic Botany 82: 143-156
- ↑ 15.0 15.1 Smetacek, V. and Zingone, A. 2013. Green and golden seaweed tides on the rise. Nature 504, doi:10.1038/nature12860
- ↑ Teichberg, M., Fox, S.E., Aguila, C., Olsen, Y.S. and Valiela, I, 2008. Macroalgal responses to experimental nutrient enrichment in shallow coastal waters: growth, internal nutrient pools, and isotopic signatures. Mar. Ecol. Prog. Ser. 368: 117–126
- ↑ Condon, R.H., Duarte, C.M., Pitt, K.A., Robinson, K.L., Lucas, C.H., Sutherland, K.R., Mianzan, H.W., Bogeberg, M., Purcell, J.E., Decker, M.B., Uye, S.-I., Madin, L.P., Brodeur, R.D., Haddock, S.H.D., Malej, A., Parry, G.D., Eriksen, E., Quiñones, J., Acha, M., Harvey, M., Arthur, J.M. and Graham W.M. 2013. Recurrent jellyfish blooms are a consequence of global oscillations. PNAS 110: 1000-1005
- ↑ Eutrophication and health. European Commission 2002. Office for Official Publications of the European Communities: Luxembourg. ISBN 92-894-4413-4.28 pp.
- ↑ 19.0 19.1 Berdalet, E., Fleming, L.E., Gowen, R., Davidson, K., Hess, P., Backer, L.C., Moore, S.K., Hoagland, P. and Enevoldsen, H. 2016. Marine harmful algal blooms, human health and wellbeing: challenges and opportunities in the 21st century. J. Mar. Biol. Assoc. U.K. 96: 61–91
- ↑ Kouakou, C.R.C. and Poder, T.G. 2019. Economic impact of harmful algal blooms on human health: a systematic review. Journal of Water and Health 17.4: 499-516
- ↑ Parsons, G.R., Morgan, A.O., Whitehead, J.C. and Haab, T.C. 2006. The Welfare Effects of Pfiesteria-Related Fish Kills: A Contingent Behavior Analysis of Seafood Consumers. Agricultural and Resource Economics Review 35(1)
- ↑ Hyytiainen, K., Hasler, B., Ericsdotter, S., Nekoro, M., Blyh, K., Artell, J., Ahlvik, L. and Ahtiainen, J. 2013. Worth it: Benefits outweigh costs in reducing eutrophication in the Baltic. BalticSTERN Summary Report for HELCOM 2013 Ministerial Meeting
- ↑ Guo, D., Wang, Y., Zhang, Y., Duan, J., Guan, F. and Hou, B. 2024. Effects of marine eutrophication environment on microbial corrosion: A review. Marine Pollution Bulletin 205, 116637
Please note that others may also have edited the contents of this article.
|