Estuarine turbidity maximum
Definition of Turbidity maximum:
Convergence zone of suspended sediment transport, where turbidity levels are high due to high suspended sediment concentrations.
This is the common definition for Turbidity maximum, other definitions can be discussed in the article
|
Contents
[hide]- 1 Suspended sediment concentration in the estuarine turbidity maximum (ETM)
- 2 Formation of the estuarine turbidity maximum
- 3 Fluid mud
- 4 Tidal flat accretion
- 5 Influence of river discharge
- 6 Infill of estuaries
- 7 Influence of dredging
- 8 'False' turbidity maxima
- 9 Environmental impact
- 10 Related articles
- 11 References
Suspended sediment concentration in the estuarine turbidity maximum (ETM)
The presence of a turbidity maximum is a common feature of estuaries with substantial tidal motion. The term turbidity maximum suggests that it corresponds to a particular location in an estuary. In fact, it is a broad zone where concentrations of fine suspended sediment are much higher than in the upstream river or in the adjacent sea, where concentrations of fine suspended sediment are generally below 100 mg/l and often even below 10 mg/l. The suspended sediment concentration (SSC) in the turbidity maximum can be very high, up to several g/l averaged over the water column. A typical example of a turbidity maximum is shown in Fig. 1. The suspended sediment concentration in the turbidity maximum depends on the supply of fine sediments from fluvial and marine sources. Erosion of ancient mud deposits in the estuary can also play a role. The most important factor, however, is the trapping efficiency of the estuary, i.e. the efficiency of hydrodynamic processes to retain fine sediments that have entered the estuary and to prevent their escape to the sea. These processes will be discussed in the next section.
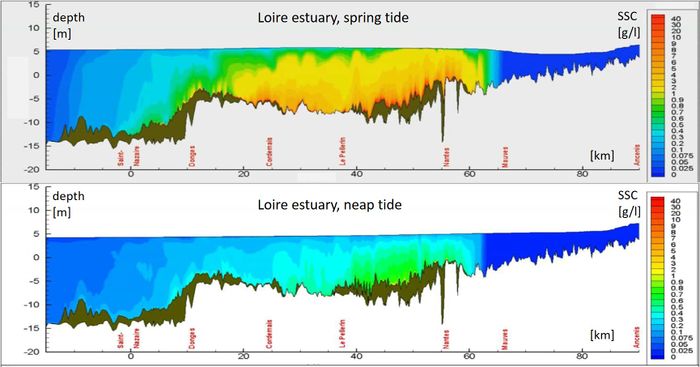
Formation of the estuarine turbidity maximum
The formation of the ETM is due to hydrodynamic processes that promote upstream (landward) transport of fine suspended sediment. For macrotidal estuaries the most important processes are[2]:
(1) Tidal asymmetry, generated by the non-linearity of tidal propagation in estuarine channels. This nonlinear tidal propagation results in shorter flood and longer ebb periods, with enhanced maximum flood currents and reduced maximum ebb currents, see Tidal asymmetry and tidal basin morphodynamics. Due to the strong dependence of sediment resuspension on flow velocity, the suspended sediment load carried by the flood currents is much higher than the suspended load carried by the ebb currents. This sediment import mechanism is sometimes designated by the term 'tidal pumping'. (It should be noted that in estuaries with substantial intertidal area, tidal asymmetry can be reversed, with stronger ebb than flood currents; however, such estuaries are more the exception than the rule.)
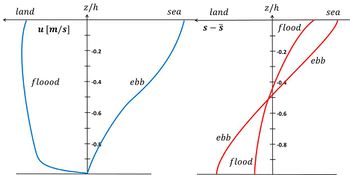
(2) Estuarine circulation, the residual flow pattern in an estuary resulting from the horizontal density difference between seawater and river water. The residual up-estuary flow along the bed of the main channel carries a higher load of suspended sediment than the compensating seaward flow near the surface, see Estuarine circulation. Estuarine circulation interacts with flood and ebb flow in different ways (see Fig. 2): the near-bed velocity gradients and shear stresses are strengthened during flood and weakened during ebb. During the flood acceleration phase, high bed shear stresses stir sediments from the bed and from the turbid near-bed layer higher into the water column[3]. This further enhances the up-estuary sediment flux compared to the down-estuary sediment flux during ebb.
(3) Stratification. Stratification is mainly due to the density difference between saline seawater and fresh river water (see Seawater density). However, in highly turbid estuaries ('hyperturbid estuaries') suspended sediment can also substantially contribute to density stratification and even dominate salinity stratification[4]. Strong stratification promotes the trapping of fluvial sediment in the estuary but reduces the import of marine sediment. An explanation is given below and in Fig. 3 (this cartoon does not include the role of the tide, tidal asymmetry and other factors influencing the ETM).
Ebb flow in estuaries is typically more stratified than flood flow. High bed shear stresses during flood promote vertical mixing. Vertical mixing during flood is also a consequence of convective overturning during flood when saline seawater is advected over less dense estuarine water[5][6][7]. During ebb, low-density river water is advected over the saline (high density) near-bed layer, thus strengthening stratification. High-turbidity estuaries become even more stratified in the phase of decelerating flood flow and at the onset of ebb flow, due to strong flocculation (the formation of macroflocs) and floc settling, which increases the density of the near-bed layer[4]. Stratification implies that estuarine outflow is mainly concentrated in the upper layer of the water column, with lower suspended sediment concentrations than in the near-bed layer. The turbid near-bed layer is eroded by the ebb flow in the surface layer, which becomes progressively thicker, carrying an increasing amount of sediment.
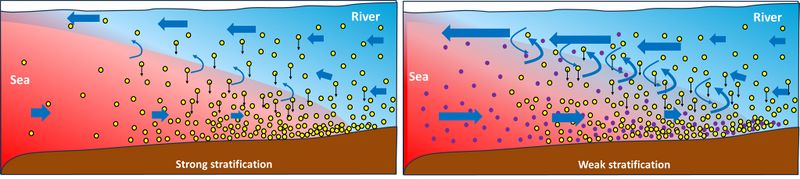
Further upstream in the estuary the residual flow is dominated by river discharge. When the ETM has not yet built up, a convergence zone of suspended sediment transport exists where downstream suspended transport by river flow is of the same order as the upstream suspended sediment transport by estuarine circulation and tidal asymmetry. In this convergence zone, fine suspended sediment accumulates and forms a turbidity maximum. The convergence zone is generally situated around the location of maximum seawater intrusion. In estuaries with strong tides, tidal asymmetry is the major mechanism for upstream sediment transport[8][9][10][11]. In this case, the highest turbidity is found upstream of the location of maximum seawater intrusion. Examples are the Ems, Gironde and Humber[12]. In these estuaries, and also in some others (e.g. Yangtze), the suspended sediment concentration in the ETM can become very high. A strong ETM strengthens density stratification and thus suppresses turbulence and frictional damping of the tidal wave. The resulting increase in tidal amplitude generates a positive feedback to ETM formation (see also next section). However, there is also a negative feedback due to the longitudinal spreading of the high-density ETM as a result of gravitational processes[13].
In microtidal estuaries, the ETM is mainly due to estuarine circulation and stratification processes; the location of the turbidity maximum is determined by the seawater intrusion length[14].
Fluid mud
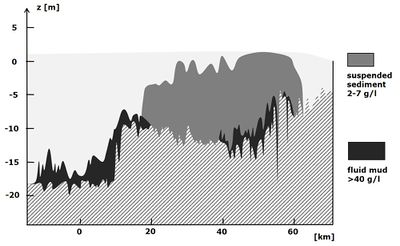
Suspended sediment settles to the channel bed during periods of slack water, especially in the neap tidal phase of the fortnightly tidal cycle. Flocculation plays an important role as an accelerator of the sedimentation process[15]. In estuaries with a strong turbidity maximum, stratification is auto-enhanced by positive feedback between sediment settling and turbulence damping at the pycnocline. This self-stratification can generate a fluid mud layer when the near-bed fine suspended sediment concentration exceeds a few tens of g/l[16] (see Fig. 4 and Dynamics of mud transport). The density (weight) of the fluid mud layer can be such that it starts flowing in down-estuary direction while the flow in the upper layer is still in flood direction (decelerating flood phase). This gravity flow opposes the classical estuarine circulation and leads to a situation where the salinity in the upper layer is higher than the salinity in the near-bed layer[4][3].
When the current velocities during spring tide become sufficiently high, the fluid mud layer is (partly) resuspended and contributes to high turbidity levels, as illustrated in Fig. 1. Resuspension at the onset of flood is due to turbulence induced by bed shear stress. Surface water is entrained into the turbulent fluid mud layer which grows while being diluted[17].
In a study of the highly turbid Ems estuary, Winterwerp et al. (2017[18]) showed that destabilization and resuspension of the fluid mud layer requires flow velocities that are greater than the internal critical flow (the celerity of the internal wave on the mud interface, which is of the order of 1-1.3 m/s). In the Ems estuary, these velocities are exceeded during flood at spring tide, except in case of high river runoff. At high river runoff, flood velocities are reduced relative to ebb velocities and not sufficiently strong to stir up the fluid mud layer. Sediment export dominates sediment import under these conditions. Suspended sediment observations along the Ems estuary suggest that not all the mud is flushed out, but that a thin consolidating mud layer is left behindCite error: Invalid <ref>
tag;
invalid names, e.g. too many.
Tidal flat accretion
Part of the fine sediments of the ETM settles on the tidal flats and marshes bordering the main estuarine channel. This reduces the volume of suspended sediment in the turbidity maximum. Estuaries with large tidal flats are also less turbid because flood dominance and the associated import of marine sediment are reduced. The ETM generally covers a wide estuarine zone because it is advected along the estuary with the tide. Tidal flats situated in this zone are subject to fast accretion. The landward part of these tidal flats that are not subject to strong wave action, will rapidly grow to a level where flooding only occurs during the highest spring tides [19][20][21][22][23], as illustrated in Fig. 5. The growth process is stimulated by the development of marsh vegetation. The volume of suspended sediment in the ETM greatly increases when it is no longer possible to store fine sediment on the mud flats, because of their elevation or because they are reclaimed[24][25]. Fast siltation also takes place in adjacent harbour basins that are situated in the high turbidity zone (see Siltation in harbors and fairways).
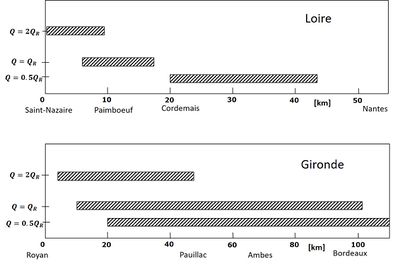
Influence of river discharge
The turbidity maximum can be flushed out of the estuary when the river discharge is sufficiently high[27][28], see Fig. 6. This happens mainly under spring tidal conditions, if the suspended sediment transport by river flow dominates transport by estuarine circulation and tidal asymmetry over the entire estuary[11]. In estuaries with such high peak discharges, the turbidity does not become as high as in estuaries where the peak discharges are lower.
Infill of estuaries
If the peak discharge is not sufficient to flush the ETM to the open sea, most of the fine sediments will remain in the estuarine mouth zone. When the river discharge decreases, these sediments are re-imported into the estuary. The estuary then acts as trap for fine sediments. In this case a gradual infill of the estuary takes place. A mud bed forms when the fine sediment concentration exceeds 1000 g/l (see Dynamics of mud transport). The infill stops when the estuarine depth and width are reduced up to a point where peak river discharges can flush the fine sediments far enough into the sea. In this way the estuary evolves towards a dynamic equilibrium.
Influence of dredging
Many estuaries are dredged for navigation purposes. Channel dredging opposes the evolution towards an equilibrium state by strengthening estuarine circulation and reducing the fluvial flushing efficiency. The enhanced fine sediment trapping leads to high concentrations in the ETM and requires a corresponding increase of maintenance dredging. A strong increase of the turbidity maxima has been observed in several estuaries (e.g., Ems, Loire) after deepening of the estuarine entrance channel[29]. See the article River tides for a more detailed discussion of the effects of channel dredging.
'False' turbidity maxima
Local elevation of suspended sediment concentrations, which may be erroneously be interpreted as a turbidity maximum, can result from interventions, for example dredging. The dumping of dredged sediment can locally increase suspended sediment concentrations to many 100s mg/l for prolonged periods of time. High river flows in the Scheldt river bring in fine sediment from beyond the weirs near Ghent, which temporarily accumulates in the upper reaches of the river, downstream of the weirs. Data would then suggest the formation of a turbidity maximum at high river flows, but obviously the underlying physical processes are different from the convergence processes explained above[30]. Turbidity maxima can also occur in the vicinity of local bed depressions that temporarily collect fluid mud[31] or at locations where turbid waters are discharged from tidal flats[32].
Environmental impact
High turbidity prevents the penetration of sunlight into the water column and therefore suppresses oxygen production by phytoplankton. At the same time, oxygen is consumed by mineralization of the degradable organic components of the fine sediments trapped in the ETM, as a result of bacterial activity or chemical oxidation [33][34][35]. This is illustrated in Fig. 7 for the Loire estuary. High turbidity causes oxygen depletion and mortality of estuarine organisms. This is a serious problem in many estuaries, particularly during warm dry periods, when rapid mineralization occurs while mixing in the water column is suppressed due to density stratification (both salinity and fine sediment concentrations contribute to stratification at small river discharge [36]). The ecological impact of the ETM is greatly exacerbated in eutrophic estuaries, see also the article Possible consequences of eutrophication.
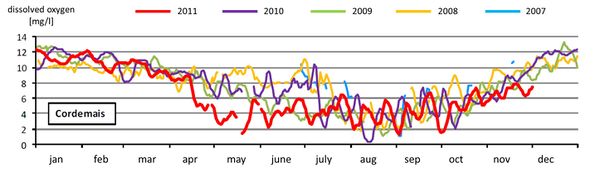
Related articles
- Estuarine circulation
- Dynamics of mud transport
- Fluid mud
- Tidal asymmetry and tidal basin morphodynamics
- Tidal rivers
- River tides
- Possible consequences of eutrophication
- Estuarine ecosystems
References
- ↑ Jump up to: 1.0 1.1 1.2 Bertier, C. 2011. Dynamique et suivi du bouchon vaseux dans l’estuaire de la Loire. Séminaire Technique sur le transport sédimentaire: Principes et expériences sur le bassin Ligrien, Vierzon 24 Novembre 2011
- Jump up ↑ Burchard, H. and Baumert, H. 1998. The Formation of Estuarine Turbidity Maxima Due to Density Effects in the Salt Wedge. A Hydrodynamic Process Study. J. Phys. Oceanography 28: 309-321
- ↑ Jump up to: 3.0 3.1 Becker, M., Maushake, C. and Winter, C. 2018. Observations of mud-induced periodic stratification in a hyperturbid estuary. Geophysical Research Letters 45: 5461–5469
- ↑ Jump up to: 4.0 4.1 4.2 Uncles, R. J., Stephens, J. A. and Law, D. J. 2006. Turbidity maximum in the macrotidal, highly turbid Humber Estuary, UK: Flocs, fluid mud, stationary suspensions and tidal bores. Estuarine, Coastal and Shelf Science 67: 30–52
- Jump up ↑ Jay, D. A. and Musiak, J. D. 1994. Particle trapping in estuarine tidal flows. J. Geophys. Res. 99: 445–461
- Jump up ↑ Prandle, D. 2004. Salt intrusion in partially mixed estuaries. Est.Coast.Shelf Sci. 59: 385-397
- Jump up ↑ Burchard, H. and Schuttelaars, H.H. 2012. Analysis of Tidal Straining as Driver for Estuarine Circulation in Well-Mixed Estuaries. J. Phys. Oceanograph. 42: 261-271
- Jump up ↑ Brenon, I. and LeHir, P. 1999. Modelling the Turbidity Maximum in the Seine Estuary (France): Identification of Formation Processes. Estuarine, Coastal and Shelf Science (1999) 49, 525–544
- Jump up ↑ Scully, M.E. and Friedrichs, C.T. 2007. Sediment pumping by tidal asymmetry in a partially mixed estuary. Journal of Geophysical Research 112, c07028
- Jump up ↑ Toublanc, F., Brenon, I. and Coulombier, T. 2016. Formation and structure of the turbidity maximum in the macrotidal Charente estuary (France): Influence of fluvial and tidal forcing. Estuarine, Coastal and Shelf Science 169: 1-14
- ↑ Jump up to: 11.0 11.1 Van Maanen, B. and Sottolichio, A. 2018. Hydro- and sediment dynamics in the Gironde estuary (France): Sensitivity to seasonal variations in river inflow and sea level rise. Continental Shelf Research 165: 37–50
- Jump up ↑ Burchard, H., Schuttelaars, H. M. and Ralston, D. K. 2018. Sediment trapping in estuaries. Annual Review of Marine Science 10: 371–395
- Jump up ↑ Zhu, C., Van Maren, D. S., Guo, L., Lin, J., He, Q. and Wang, Z. B. 2021. Effects of sediment-induced density gradients on the estuarine turbidity maximum in the Yangtze Estuary. Journal of Geophysical Research: Oceans 126, e2020JC016927
- Jump up ↑ Restrepo, J.C., Schrottke, K., Traini, C., Bartholomae, A., Ospino, S., Ortíz, J.C., Otero, L. and Orejarena, A. 2018. Estuarine and sediment dynamics in a microtidal tropical estuary of high fluvial discharge: Magdalena River (Colombia, South America). Marine Geology 398: 86–98
- Jump up ↑ Guo, C., He, Q., Guo, L. and Winterwerp, J.C. 2017. A study of in-situ sediment flocculation in the turbidity maxima of the Yangtze Estuary. Estuarine, Coastal and Shelf Science 191: 1-9
- Jump up ↑ Winterwerp, J.C. 2016. Fine sediment transport by tidal asymmetry in the high-concentrated Ems River: indications for a regime shift in response to channel deepening. Ocean Dynamics 61: 203–215
- Jump up ↑ Bruens, A.W., Winterwerp, J.C. and Kranenburg, C. 2012. Physical and numerical modeling of the entrainment by a high-concentration mud suspension. ASCE, J. Hydraul. Eng. 138: 479–490
- Jump up ↑ Winterwerp, J.C., Vroom, J., Wang, Z-B., Krebs, M., Hendriks, E.C.M., van Maren, D.S., Schrottke, K., Borgsmüller, C. and Schoel, A. 2017. SPM response to tide and river flow in the hyper-turbid Ems River. Ocean Dynamics 67: 559–583
- Jump up ↑ Allen, J.R.L. 2000. Morphodynamics of Holocene salt marshes: a review sketch from the Atlantic and Southern North Sea coasts of Europe. Quaternary Science Reviews 19: 1155-1231
- Jump up ↑ Temmerman, S., Govers, G., Meire, P. and Wartel, S. 2003. Modelling long-term tidal marsh growth under changing tidal conditions and suspended sediment concentrations, Scheldt estuary, Belgium. Marine Geology 193: 151-169
- Jump up ↑ French, J. 2006. Tidal marsh sedimentation and resilience to environmental change: Exploratory modelling of tidal, sea-level and sediment supply forcing in predominantly allochthonous systems. Marine Geology 235: 119–136
- Jump up ↑ Deloffre, J., Verney, R., Lafite, R., Lesueur, P., Lesourd, S. and Cundy, A.B. 2007. Sedimentation on intertidal mudflats in the lower part of macrotidal estuaries: Sedimentation rhythms and their preservation. Marine Geology 241:19–32
- Jump up ↑ Boyd, B.M., Sommerfield, C.K., Elsey-Quirk, T. 2017. Hydrogeomorphic influences on salt marsh sediment accumulation and accretion in two estuaries of the U.S. Mid-Atlantic coast. Marine Geology 383: 132–145
- Jump up ↑ Van Maren, D.S., Oost, A.P., Wang, Z.B. and Vos, P.C. 2016. The effect of land reclamations and sediment extraction on the suspended sediment concentration in the Ems Estuary. Marine Geology 376: 147–157
- Jump up ↑ Winterwerp, J., Wang, Z.B., van Braeckel, A., Holland, G. and Koesters, F. 2013. Man-induced regime shifts in small estuaries - II: A comparison of rivers. Ocean Dynamics 63: 1293-1306
- Jump up ↑ Sottolichio, A. and Castaing, P. 1999. A synthesis on seasonal dynamics of highly concentrated structures in the Gironde estuary. Comptes Rendus Acad. Sci. Earth Planet. Sci. 329: 795–800
- Jump up ↑ Jalon-Rojas, I., Schmidt, S., Sottolichio, A. and Bertier, C. 2016. Tracking the turbidity maximum zone in the Loire Estuary (France)based on a long-term, high-resolution and high-frequency monitoring network. Continental Shelf Research 117: 1–11
- Jump up ↑ Pritchard, M. and Green, M. 2017. Trapping and episodic flushing of suspended sediment from a tidal river. Continental Shelf Research 143: 286–294
- Jump up ↑ Van Maren, D.S., Kessel, T., Cronin, K. and Sittoni, L. 2015. The impact of channel deepening and dredging on estuarine sediment concentration. Continental Shelf Research 95: 1–14
- Jump up ↑ Vandenbruwaene, W., Vanlede, J., Plancke, Y., Verwaest, T. and Mostaert, F. 2015. Slibbalans Zeeschelde: Deelrapport 4 – Historische evolutie SPM. Versie 4_0. WL Rapporten, 00_029. Waterbouwkundig Laboratorium & Antea: Antwerpen, België (in Dutch)
- Jump up ↑ Schrottke, K., Becker, M., Bartholomae, A., Flemming, B.W. and Heebeln, D. 2006. Fluid mud dynamics in the Weser estuary turbidity zone tracked by high-resolution side-scan sonar and parametric sub-bottom profiler. Geo-Mar Letters DOI 10.1007/s00367-006-0027-1
- Jump up ↑ Doxaran, D., Froidefond, J-M., Castaing, P. and Babin, M. 2009. Dynamics of the turbidity maximum zone in a macrotidal estuary (the Gironde, France): Observations from field and MODIS satellite data. Estuarine, Coastal and Shelf Science 81: 321–332
- Jump up ↑ Morris, A. W., Loring, D. H., Bate, A. J., Howland, R. J. M., Mantoura, R.F. C. and Woodward, E. M. S. 1982. Particle dynamics, particulate carbon and the oxygen minimum in an estuary. Oceanol. Acta 5: 349-353
- Jump up ↑ Uncles, R.J., Joint, I. & Stephens, J.A. 1998. Transport and retention of suspended particulate matter and bacteria in the Humber-Ouse Estuary, United Kingdom, and their relationship to hypoxia and anoxia. Estuaries 21: 597–612
- ↑ Jump up to: 35.0 35.1 Etcheber, H., Taillez, A., Abril, G., Garnier, J.A., Servais, P., Moatar, F. and Commarieu, M-V. 2007. Particulate organic carbon in the estuarine turbidity maxima of the Gironde, Loire and Seine estuaries: origin and lability. Hydrobiologia 588: 245–259
- Jump up ↑ Winterwerp, J.C. 2006. Stratification effects by fine suspended sediment at low, medium, and very high concentrations. Journal of Geophysical Research 111, c05012
Please note that others may also have edited the contents of this article.
|