Deep sea habitat
This article describes the habitat of the deep sea. It is one of the sub-categories within the section dealing with biodiversity of marine habitats and ecosystems. It gives an overview about the characteristics, the bottom topography, the adaptations to the environment of the biota and the threats.
Contents
Introduction
The deep sea floor represents the largest habitat on earth. It ranges from the edge of the continental shelf at about 200m depth to the bottom of the ocean. At the edge of the continental shelf is the shelf break, where the gradient of the floor increases down the continental slope. Below the continental slope lies a large sedimentary deposit, the so-called continental rise. At around 4,000 meters depth, the ocean floor is reached and extends over the ocean basins at depths of 5,000 metres on average. This is called the abyssal plain. The zone between the continental shelf and the abyssal plain is the bathyal zone. In some places, the sea floor drops again into elongated trenches with depths of 10 to 11 kilometres. This region is the hadal region. The ocean floor is interrupted by mountain chains known as the mid-oceanic ridge systems. Other features on the ocean floor are seamounts, trenches and hydrothermal vents.[1]
Characteristics
The temperature of the waters of the deep sea varies from 4°C to -1°C. Exceptions are the Mediterranean Sea with an average temperature of 13°C in the upper 4,000 metres and the Red Sea where the bottom water can reach temperatures up to 21.5°C at depths of 2,000 metres. The lowest temperatures of about -1.9°C are found in the Antarctic Ocean. The salinity of the seawater is relatively constant. Below 2,000 metres, the seawater salinity is close to 35 (34.8 ± 0.3) PSU and at the very deepest layers it is 34.65. The oxygen concentrations are near saturation except in the oxygen minimum layer below the photic zone, between about 200 - 1500 m depth. This is related to oxygen consumption by microbial mineralization of organic matter sinking out of the photic zone (see Possible consequences of eutrophication). The pressure in the deep ocean is very high; it increases by 1 bar ([math]=10^5[/math] Pa, close to 1 atmosphere) per 10 meter increase in depth.[2] In the deep ocean, light is almost absent. It is a dark environment and the organisms are adapted to it. The combination of limited food and oxygen penetration at depth in sediments confines the vast majority of organisms to the upper few centimeters of sediment near the sediment-water interface. [3]
Ocean waters have a decreasing saturation state of calcium carbonate [math]CaCO_3[/math] at greater depth, due to the greater solubility of [math]CO_2[/math] and lower [math]pH[/math] (higher acidity) at low temperature and high pressure. This explains why calcifying organisms do not occur at great depths in the ocean (below 4000-5000 m), see Ocean acidification.
The circulation in the deep sea is dealt with in the article Ocean circulation.
Adaptations
Finding food and avoiding predation require special adaptations for the animals of the deeper layers of the water column where there is no plant growth at all. Many mid-water animals often have bizarre body shapes, because they never come into contact with a physical barrier. The most common form is more or less spherical, often with long and delicate extensions. Most animals do not need a rigid skeleton because they spend all their time suspended in water. An important adaptation is not to be seen too easily by potential predators. In the upper parts of the mesopelagic zone, where there is still some sunlight, many animals (e.g. gelatinous zooplankton, heteropod molluscs, arrow worms, certain amphipods, and a variety of decapod shrimps) have more or less transparent bodies. Full transparency is the extreme case, as it requires polarization of an organism's molecules as well as behavioral modifications to blend in. An alternative is to have silver sides that act as mirrors and luminiscent organs along the bellies that hide the bodyshape (e.g. hatchetfishes of the genus Argyropelecus). At deeper layers, the organisms have a dark red, black or brown colour. These colours do not reflect the most usual blue-green colour of bioluminescence. In these deeper waters, the anglerfish has a fishing rod with bioluminescent lures to attract preys. In the mid-water, many fishes have big teeth and big mouths to take up as much as possible. Dial vertical migration is another adaptation of many animals. The general pattern is that animals spend the daylight hours at depth and move upwards during the night. This is to avoid predation and to feed. Bioluminescence is another adaptation. Depending on the depth, organisms use it as camouflage to protect them from predators or to confuse or frighten predators rather than to hide from them. Other animals use it to look for a potential mate. A few deep-sea animals use it together with another light organ that produces deep red light. Other animals cannot see this red light, but the red light will be reflected very efficiently by the red bodies of the specific prey. The red light is a recognition mark for other members of the same species. An example of such an organism is the dragonfish Malacosteus (Fig. 2). [4][5]
Biota
The mid-water communities include representatives of most major animal groups on Earth, from tiny single-celled organisms only a fraction of a millimetre, through all the invertebrate phyla, to fish several meters long. Most of them have an average size in the range of centimetres. The dominant species are crustaceans, fish and a variety of animals with soft and jelly-like bodies such as jellyfish.
The deep-sea floor is covered with many mounds and depressions formed by benthic animals such as worms, mollusks, crustaceans, starfish, brittlestars, shrimps, fishes sea cucumbers and sea urchins (Fig. 3). Because the deep-sea floor is poor in food resources, it cannot support large populations of large animals. This causes large animals to be spread over a greater range than elsewhere. For this reason, many animals adopt a ‘sit and wait’ strategy. This strategy means that the animals stay at one place and wait until food is falling down or carried to them with the currents. These animals are sponges, sea anemones, tube worms, barnacles and other smaller animals.[5]
![]() Fig. 3a. Squid with many light organs [6] |
![]() Fig. 3b. Deep-sea bottom with fish [6] |
![]() A gorgonian feeding on a basketstar [6] |
There are five groups that are dominant in terms of numbers. These are: foraminiferans, radiolaria, nematodes, crustaceans (amphipod shrimps, isopods, copepods, bivalves) and polychaete worms (Fig. 4).
![]() Fig. 4a. Foraminifera [6] |
![]() Fig. 4b. Radiolaria [7] |
![]() Fig. 4d. Isopod [8] |
Giant animals and strange creatures are common in the deep sea. These are sometimes called monsters of the sea. There are a lot of examples. Many myths of monsters are based on sightings of squid. Sharks are also seen as monster and aggressive animals.
Other animals can be seen as monsters because they are very large in comparison to the species that we know. An example is the giant isopod. This phenomenon is called gigantism (Fig. 5)
![]() Fig. 5b. Giant squid [6] |
![]() Fig. 5d. Sea spider [6] |
Mid-oceanic ridges
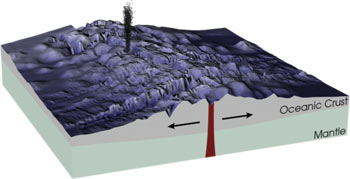
A mid-oceanic ridge (Fig. 6) is a submarine mountain range formed by plate tectonics. It is a characteristic of the boundary between two diverging tectonic plates. The ridge results from convection currents which rise in the mantle as magma and emerge as lava, creating the new crust upon cooling. The mid-oceanic ridges are connected and form a single global mid-oceanic ridge system that is part of every ocean. It can be occupied by a rift valley that is formed by geological forces that pulled apart and broke the solid rock - a process called faulting. Mid-ocean ridges are active features characterized by frequent, shallow earthquakes, many faults and widespread volcanism. It is also segmented by transform faults, where fractured rocks slide past one another. This causes a zigzag pattern. At many places, hydrothermal vents and cold seeps are situated on these mid-oceanic ridges. [9]
Hydrothermal vents
The hydrothermal vents (Fig. 7) are associated with parts of the ocean floor that exhibit high levels of tectonic activity such as mid-oceanic ridges. In these regions, hot magma chambers below the seabed heat up water that has permeated into the ocean floor. Vents occur where tectonic spreading and subduction create fissures in the Earth’s crust, allowing sea water to percolate through the crust where the mantle heats it. Water that seeps into cracks of the crust first loses minerals (oxygen, potassium, magnesium, ..) but is later charged with new chemicals (copper, zinc, iron, hydrogen sulfide, ..) when the highest temperatures (350-400°C) are reached. Water rich in minerals and reduced compounds such as hydrogen sulfide later percolates out through the crust at extremely high (200C–400C) temperatures; extreme pressure prevents it from boiling. It cools quickly, however, as it mixes with ambient sea water. The 'smoke' is formed by black metal-sulfide minerals when the hot fluid rises up and is released in the cold, oxygen-rich seawater. [3][10] This type of very hot hydrothermal vents is called a black smoker. They are islands in a sea of mud. The mixture of sedimentary and hard substrate habitats that characterize vent fields often support a large biomass of specialized biota. Cooler vents also exist; they are called white smokers. [3]
The biomass of organisms associated with the hydrothermal vents is generally huge. Their food supplies are adapted to the extreme conditions and the absence of sunlight. Chemoautotrophic bacteria and archaea that live freely or form symbioses within specialized tube worms, clams, and mussels, drive the high productivity at hydrothermal vents and seeps. These bacteria and archaea can tolerate extremely high temperatures (called hyperthermophiles (80-115°C) and superthermophiles (>115°C)). They synthesize organic compounds by oxidizing hydrogen sulfide or methane from the vents. The bacteria are prey to larger organisms, primary consumers such as sea anemones, shrimps, crabs and fish. Bacteria are also often symbionts, in which case they pass the organic compounds directly to the hosts. These hosts often have reduced guts and little or no feeding structures. Both symbiotic partners benefit in that the host provides a physically stable habitat in the immediate proximity of hydrogen sulfide and the bacteria provide a rich food supply to the host. [3]
The tube worm Riftia pachyptila, living on the floor of the Pacific Ocean near hydrothermal vents, is a typical example of host-symbiont relationship. It has a red plume of gills sticking out of the top of the worm’s white tubular bodies and can reach a length of 3 m (Fig. 7). These animals feed on organic compounds produced by symbiotic sulfur bacteria living in their tissues. They are also found around cold hydrocarbon seeps. The worm has no mouth or gut and it has special chemosynthetic bacteria in the tissues of its body, called the trophosome. The chemicals that the bacteria need are supplied by the worm's blood.[4] The extreme temperature gradients and toxic concentrations of hydrogen sulfide create a habitat that few organisms can tolerate, so relatively few species can utilize such symbiotic relationships.
Vents usually have a restricted distribution on any given ridge segment and persist for about 10 to 20 years, until there is local extinction of the vent community. Animals colonize new vents rapidly, grow fast, and produce enough offspring to colonize the next vent. In comparison with the rest of the deep sea, few species have adapted to the extreme thermal (4°C up to temperatures in excess of 150°C) and chemical (high concentrations of cadmium, lead, cobalt, and arsenic) conditions at hydrothermal vents.[11] Ecosystems associated with hydrothermal vents such as black smokers or white smokers, so-called hydrothermal communities, can nevertheless be hundreds of times more abundant than communities on the adjacent sea floor.[1]
Cold seeps

Cold seeps (Fig. 8) occur over fissures on the seafloor caused by tectonic activity and are found along active and passive continental margins. They are related to geological processes such as tectonically induced high-fluid pressures, petroleum or natural gas escape, catastrophic erosion and slides. Methane-rich fluids of thermogenic and/or biogenic origin are a major source of energy. Methanotrophic bacteria use methane to produce carbonates by methane reduction. The carbonates can react with calcium to form calcium carbonate. Production of sulfide by sulfate reduction provides another energy source. Sulfate reducing bacteria use sulfate to form sulfides and this sulfide is oxidized by sulfur oxidizing bacteria that release sulfate again into the seawater. Macro-invertebrates such as mussels and tubeworms thrive on these chemosynthetic microorganisms.
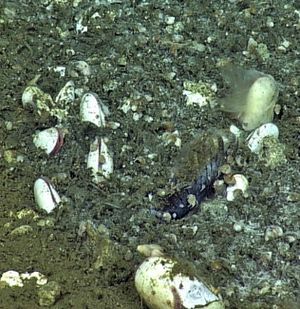
Cold seeps have a lower metazoan biodiversity than hydrothermal vents but a higher productivity. This is because of the high biomass of megabenthic organisms. The communities are often dominated by bivalves that have symbiotic relationships with chemosynthetic bacteria. Organisms that can be found here are tube worms, bivalves, crabs, anemones and soft corals. An example of an organism is the vesicomyid clam (Fig. 9) that has a symbiotic relationship with sulfur oxidizing bacteria.
Mud volcanoes and seamounts
- A mud volcano or mud dome refers to formations created by geo-excreting fluids and gasses. The most abundant gas that is released is methane.
- Knolls are submarine hills several hundred meters high.
- Seamounts are mountains rising more than 1000 m from the ocean floor without reaching the water’s surface.
Seamounts are typically formed from extinct volcanoes, which have risen abruptly. It is estimated that there are more than 30,000 seamounts in the ocean (Fig. 10), but only few have been studied. They are hotspots of marine life. Their steep topography can generate localized eddies and upwelling zones. These currents create areas of enhanced primary productivity and associated high concentrations of zooplankton that provide food for fish. Benthic animals that occur in dense concentrations feed on planktonic particles suspended by the currents. Marine mammals such as sharks, tuna and cephalopods all gather over the seamounts to feed. Even seabirds appear to be more abundant in the zones with shallow seamounts.[13].
Volcanic rock dominates the substrate, but sediments can be present if the top of the seamount is flattened to form a guyot or if the seamount has gently sloped flanks. Because seamounts can reach high above the seafloor (1000s of meters), the fauna often differs from that on the surrounding abyssal plain. The hard substrate supports a greater variety of species than sedimentary environments. In some cases, seamounts in the Pacific Ocean extend to the oxygen minimum layer; these low-oxygen conditions favor a very different set of species compared to habitats with abundant dissolved oxygen.[3]

Trenches
Trenches, the deepest ocean habitats, form where one ocean plate subducts beneath another. Relatively steep sides contribute to poor circulation and occasional mud slumping that, along with limited food supply, make trenches inhospitable to most organisms. Representatives from most marine taxa have been observed in trenches, with gastropods, polychaetes, bivalves and holothurians occurring to 11,000 m, though fishes are also present to c. 8200 m. Due to the relatively isolated nature of trenches, rates of endemism within trenches appear to be high, with different trenches harboring relatively high numbers of unique species.[5]
Sinking organic matter

Carcasses of large animals sink from the overlying water column to the bottom (Fig. 11). They produce many localized food hot-spots. The carcass sinks slowly at first, but as the pressure increases the carcass will gather speed. It may take many hours to reach the bottom and it may be attacked during the downward journey. When a carcass reaches the bottom, it attracts amphipods within minutes. These crustaceans have powerful jaws, which can slice flesh. They can detect the odor of a carcass carried along with the water currents. After them, several fishes arrive and feed on the carcass. Shrimps can also occur on it. When these animals have eaten enough or when there is nothing suitable left for them, the remainders will attract slow-moving animals such as mollusks, starfish, brittlestars and sea cucumbers. Finally, bacteria will finish the job. They can even break down the bones. Within weeks the carcass is cleared away.
Aggregates of organic particles (mostly detritus) also fall through the water column as so-called 'marine snow'. Particles floating in the water are glued together by ubiquitous extrapolymeric substances exuded by marine organisms. These aggregates grow over time and may reach several centimeters in diameter, traveling for weeks before reaching the ocean floor. If they reach the seafloor before being mineralized, they generally accumulate in thick patches separated by relatively barren areas. This export of organic matter to deep ocean water layers and the ocean floor is an important carbon sequestration mechanism, called 'ocean's biological pump'.
Threats
Human activities can cause serious damage to the deep sea. Many deep-sea organisms grow very slowly, reproduce at an older age than their shallow-water counterparts, and produce very few offspring per individual. They are therefore extremely vulnerable to disturbance and habitat damage. Examples of human activities that have a negative impact are [15]:
- Fishing activities: overfishing, bycatch or incidental mortality of non-target species, destructive practices and illegal, unreported and unregulated fishing activities (IUU) (see also Overexploitation). Deep-sea fisheries utilize trawls and dredges that damage the integrity of the benthic habitat, injure organisms, and remove many non-target species as by-catch. Fishing effort outpaces the capacity of the population to recover. Non-target species are removed as by-catch or injured by fishing gear.
- Noise pollution such as powerful sonar systems and airguns.
- Shipping: noise, accidental spills of oil, discharge of garbage, oily wastes, sewage, chemical residues and ballast water, anti-fouling products.
- Installation of submarine cables and pipelines and disturbance by deep sea mining.
- Waste disposal encompasses materials ranging from sewage sludge to radioactive waste. Pollutants already occur in deep sea organisms at low concentrations.
- Effects of climate change may not be obvious for decades to hundreds of years from now, but warming and ocean acidification may have a great impact.
- Disturbance of hydrothermal vent ecosystems by the collection of thermophilic (“heat loving”) bacteria that have generated great interest for their bioactive compounds.
Related articles
References
- ↑ 1.0 1.1 Kaiser M. et al. 2005. Marine ecology: Processes, systems and impacts. Oxford University Press. p.584
- ↑ Gage J.D. and Tyler P.A. 1991. Deep-sea biology – A natural history of organisms at the deep-sea floor. Cambridge University Press. p. 504
- ↑ 3.0 3.1 3.2 3.3 3.4 Snelgrove, P.V.R. and Grassle, J.F. 2019. Deep-Sea Fauna. In: Encyclopedia of Ocean Sciences, 3rd Edition, Vol. 1 Elsevier. pp. 706-714
- ↑ 4.0 4.1 Rice T. 2000. Deep Ocean. The natural history museum, London. p. 96
- ↑ 5.0 5.1 5.2 Snelgrove, P.V.R. and Grassle, J.F. 2019. Deep-Sea Ecology. In: Encyclopedia of Ocean Sciences, 3rd Edition, Vol. 1 Elsevier. pp. 35-45
- ↑ 6.0 6.1 6.2 6.3 6.4 6.5 6.6 6.7 Photo credit NOAA
- ↑ Photo credit Wim Van Egmont
- ↑ Photo credit Chris Perridas
- ↑ 9.0 9.1 http://en.wikipedia.org/wiki/Mid-oceanic_ridge
- ↑ http://www.divediscover.whoi.edu/vents/vent-chemistry.html
- ↑ Grassle, J.F. 2001. Marine ecosystems. Encyclopedia of Biodiversity, Volume 4. Academic Press
- ↑ https://interactiveoceans.washington.edu/05/2023/vesicomyid-clam/
- ↑ Rogers, A. D. 1994. The biology of seamounts. Advances in Marine Biology 30: 305–350
- ↑ Yesson, C., Clark, M.R., Taylor, M. and Rogers, A.D. 2011. The global distribution of seamounts based on 30-second bathymetry data. Deep Sea Research Part I: Oceanographic Research Papers 58: 442-453. DOI: http://dx.doi.org/10.1016/j.dsr.2011.02.004.
- ↑ UNEP Regional Seas Report and Studies No.178. 2006. Ecosystems and Biodiversity in Deep Waters and High Seas. p. 60
Please note that others may also have edited the contents of this article.
|