Difference between revisions of "Ocean circulation"
Dronkers J (talk | contribs) |
|||
(16 intermediate revisions by 2 users not shown) | |||
Line 1: | Line 1: | ||
− | This article gives an | + | |
− | + | {{Review | |
+ | |name=Job Dronkers | ||
+ | |AuthorID=120 | ||
+ | }} | ||
+ | |||
+ | |||
+ | |||
+ | |||
+ | This article gives an introduction to the main circulation patterns in the ocean. Ocean circulation patterns are strongly influenced by the Earth's rotation as explained in the articles [[Ekman transport]] and [[Geostrophic flow]]. | ||
==Introduction== | ==Introduction== | ||
− | + | By redistributing heat over the globe, [[Open oceans|ocean]] currents have a major impact on the global climate. They cause the relative mildness of the Western European climate, for example. Ocean and atmospheric currents form a coupled dynamic system. Instabilities of this system, the El Nino Southern Oscillation (ENSO) in particular, produce important climate fluctuations. Ocean currents not only distribute heat, but they also play a crucial role in the global ecosystem by storing <math>CO_2</math> and recycling nutrients. | |
+ | |||
+ | |||
+ | ==Currents== | ||
+ | |||
+ | There are two main types of ocean currents: currents driven mainly by wind and currents mainly driven by [[Seawater density|density]] differences. Density depends on temperature and [[salinity]] of the water. Cold and salty water is dense and will sink. Warm and less salty water will float. Although tides are generally a dominant driver of water motion in shallow coastal waters, their relative importance in the oceans is less. It should be noted, however, that tides are mainly generated in the oceans (by the gravitational forces of moon and sun) and are amplified when propagating onto the continental shelf (see the article [[Ocean and shelf tides]]). | ||
+ | |||
+ | |||
+ | ==Wind-driven ocean currents== | ||
+ | |||
+ | ===Global wind field=== | ||
+ | The large-scale global wind field consists of dominating westerly winds at latitudes between 30 and 60 degrees in the northern and southern hemispheres (the ''Westerlies'') and dominating easterly winds in the tropical/subtropical zone (the ''Trade winds''). This wind field pattern results from the low atmospheric pressure in the tropics (warm ascending air) and high atmospheric pressure in the subtropics (cooled descending air). The near-surface air flow - toward the equator at low latitudes and toward the poles at high latitudes (so-called ''Hadley cells'') - is deflected by [[Coriolis acceleration|earth rotation]], hence giving rise to the ''Westerlies'' and the ''Trade winds''. | ||
+ | |||
+ | ===Surface currents=== | ||
+ | Wind stress generates strong currents (up to several m/s) in the ocean surface layer. The thickness of the surface layer entrained by wind is of the order of 500 meters (about the thickness of the thermocline at low- and mid-latitudes), up to a maximum of 2000 m. Due to [[Coriolis acceleration|earth rotation]] the main ocean current system consists of large anticyclonic [[Gyre|gyres]] (clockwise rotating in the northern hemisphere and anticlockwise in the southern hemisphere) <ref> Munk, W. H. 1950. On the wind-driven ocean circulation. J. Met. 7, 79-93.</ref>. There are five major [[Gyre|gyres]]: the North Atlantic, the South Atlantic, the North Pacific, the South Pacific and the Indian Ocean [[Gyre]], see figure 1. | ||
+ | The ''Antarctic Circumpolar Current'' is situated in the Southern Ocean and constantly circles around Antarctica because there are no land masses to interrupt the currents. It is an eastward-flowing current driven by the dominant western winds at this latitude. | ||
+ | |||
+ | |||
+ | [[image: Ocean-Currents gkplanet.jpg |center|thumb|800px|Caption|Fig. 1. Ocean surface currents <ref>https://commons.wikimedia.org/wiki/File:Corrientes-oceanicas-en.svg</ref>]] | ||
+ | |||
+ | The most famous ocean current, the ''Gulf Stream'', is a vast moving mass of water, transporting an enormous amount of heat from the Caribbean across the ocean to Europe. It passes by the US east coast as a narrow jet, due to the northward increase of the [[Coriolis acceleration]] <ref>Stommel, H. 1948. The westward intensification of wind-driven ocean currents. Transactions, American Geophysical Union, 29: 202-206.</ref> and then spreads out as a meandering current over the ocean while generating a series of meso-scale eddies and whirls. The North Atlantic Gyre is completed by the ''Canary Current'' in the Eastern Atlantic that transports relatively cold water south and west. The ''Kuroshio'' is a warm boundary current in the north-western Pacific, similar to the ''Gulf Stream''. It is part of the large gyre formed by the ''California Current'' and the ''North Equatorial Current''. The ''North Equatorial Current'' and ''South Equatorial Current'' are driven by the easterly trade winds over the Pacific. The Southern Pacific Gyre is completed by the warm ''West Australian Current'' and the cold ''Peru Current''. | ||
− | |||
− | + | ===Upwelling=== | |
− | |||
+ | [[image:EkmanTransport.jpg|left|thumb|250px|Caption|Fig. 2. Principle of coastal upwelling, see the article [[Ekman transport]].]] | ||
− | [[image: | + | [[image:Equatorial upwelling.jpg|thumb|right|200px|Caption|Fig. 3. [[Ekman transport]] and resulting equatorial upwelling, with rise of the thermocline <ref>http://www-das.uwyo.edu/~geerts/cwx/notes/chap11/equat_upwel.html</ref>]] |
+ | In regions where [[Ekman transport]] deflects the boundary current from the coast, water from the deep ocean rises to the ocean surface, see figure 2. This phenomenon is called 'upwelling' and is very important for enrichment of surface waters with organic matter and nutrients. Upwelling zones are characterized by a very rich marine life with abundant resources for fishery. Upwelling zones exist at the southward flowing boundary currents in the Northern Hemisphere (''California Current'' along the US West Coast, ''Canary Current'' along the West African coast) and at the northward flowing boundary currents in the Southern Hemisphere (''Peru Current'' along the South American West Coast and ''Benguela Current'' along the South African West Coast). | ||
− | |||
− | |||
− | [[ | + | Upwelling also occurs at the equator at the Pacific Ocean (''Equatorial upwelling''). The ''North Equatorial Current'' is deflected to the north and the ''South Equatorial current'' to the south as a consequence of the Coriolis effect. This produces upwelling of nutrient rich water and cooling of the surface water near the equator of the Pacific, see figure 3. Downwelling zones exist north and south of the equator, as explained in the article [[Ekman transport]]. |
+ | |||
+ | |||
+ | ===El Nino Southern Oscillation=== | ||
+ | Instability of the coupled ocean-atmosphere dynamics produces large fluctuations in the climate of the Pacific region, which are felt at the global scale. Weakening of the easterly trade winds allows warm water from the Western Pacific to flow back with the ''Equatorial Counter Current'' to the eastern South American boundary, where upwelling currents of cold deep ocean water are shut off. This results in relative warming of the Eastern Pacific (lowering the sea surface atmospheric pressure) and relative cooling of the Western Pacific (increasing the sea surface atmospheric pressure) and hence induces a further weakening of the easterly trade winds. This feedback strengthens the so-called ''El Nino'' phase of the oscillation <ref> Bjerknes, J. 1969. Atmospheric teleconnections from the equatorial Pacific. Mon Weather Rev. 97:163–172.</ref><ref> Wyrtki, K. 1973. Teleconnections in the Equatorial Pacific Ocean. Science 180: 66-68.</ref>. The shut-off of the food-rich upwelling currents has major consequences for marine life and fisheries. <ref>Rice T. 2000. Deep Ocean. The natural history museum, London. </ref>. After a number of years (three on average, but variable) the system sweeps back to the opposite phase, called ''La Nina''. The onset and offset of the oscillation are still not fully understood. | ||
− | ==Deep circulation== | + | ==Deep ocean circulation== |
− | + | [[Thermohaline circulation of the oceans|Deep ocean circulation]] is primarily driven by density differences. It is called ''thermohaline circulation'', because density differences are due to temperature and salinity. Density differences are small and the flow velocity is low, of the order of a few cm/s. However, the water masses moving around by thermohaline circulation are huge. Water fluxes are of the order of 20 Sv or more (Sv = Sverdrup = 1 million m<sup>3</sup>/s). Density gradients alone are not sufficient for sustaining the deep ocean circulation. Upwelling and mixing processes, to bring deep ocean water back to the surface, are required too <ref name=S>Rahmstorf, S. 2006. Thermohaline Ocean Circulation. In: Encyclopedia of Quaternary Sciences, Edited by S. A. Elias. Elsevier.</ref>. | |
+ | ===Deep water formation=== | ||
+ | The [[Seawater density|density]] of surface water increases when frigid air blows during winter across the ocean at high latitudes. The water density increases further by evaporation and by salt expulsion when sea ice is formed. Deep ocean water masses are formed in the Arctic and Antarctic regions by sinking of dense water with a temperature less than 4°C from the surface to great depth. From these regions, a cold deep water layer spreads over the entire ocean basins. | ||
− | + | ===Conveyor belt=== | |
− | |||
− | + | [[image:AMOC.jpg|thumb|left|400px|Caption|Fig. 4. Schematic representation of the Atlantic Meridional Overturning Current.]] | |
− | |||
− | + | The thermohaline circulation moves water masses around between the different ocean basins <ref> Wüst, G. 1968. History of investigations of the longitudinal deep-sea circulation (1800–1922). Bulletin de l’Institut Oceanographique, Monaco, Numero Special 2, 109–120.</ref><ref> Stommel H. and Arons, A.B. 1960. On the abyssal circulation of the world ocean—II. An idealized model of the circulation pattern and amplitude in oceanic basins. Deep-Sea Research 6: 217–233.</ref>. 'Ocean conveyor belt' is the popular name of this inter-basin circulation. The conveyor belt is fed in the northern North Atlantic with high-salinity water (due to evaporation) supplied by the ''Gulf Stream'', which sinks to great depth after cooling down in the Arctic region, forming the ''North Atlantic Deep Water'' (NADW). The replacement of this dense sinking water generates a continuous surface flow feeding the conveyor belt. The NADW flows from the Arctic region southward, as a deep boundary current along the American shelf <ref> Stommel H., Arons, A.B. and Faller, A.J. 1958. Some examples of stationary flow patterns in bounded basins. Tellus 10 (2): 179–187.</ref>. This current compensates for the net northward surface flow in the Atlantic Ocean. This circulation along the north-south axis is called ''Atlantic Meridional Overturning Circulation'' (AMOC) , see figure 4. | |
− | |||
− | |||
− | |||
− | |||
− | = | + | The NADW finally joins the ''Antarctic Circumpolar Current'' and enters the Indian and Pacific oceans. The cold dense water from the Antarctic zone fills the deep water layer in these oceans and then gradually rises and mixes with the surface waters of the Indian and Pacific oceans. The mixing of deep ocean water is promoted by strong surface winds, by tides, by upwelling and by abyssal circulation<ref> Stommel H. 1958. The abyssal circulation. Deep-Sea Research 5 (1): 80–82.</ref><ref name=S></ref>. The circulation is finally completed by a warm surface return current to the Atlantic Ocean that passes south of Africa and America, see figure 5. The whole trip takes more than 1,000 years to complete. |
− | |||
+ | |||
+ | [[image:ConveyorBelt_Broecker_Maier-Reimer.jpg|thumb|center|500px|Caption|Fig. 5. Simplified scheme of the global thermohaline circulation, adapted from Broecker (1991) <ref>Broecker, W.S. 1991. The Great Ocean conveyor. Oceanography 4: 79–89</ref>.]] | ||
+ | |||
+ | |||
+ | |||
+ | ===Energy for maintaining the large-scale thermohaline circulation=== | ||
+ | To maintain the large-scale thermohaline circulation of the ocean, it has been estimated that about 2.1 TW (<math>10^{12}</math> Watts) of mixing energy is required (Munk and Wunsch, 1998) <ref>Munk, W. H., and Wunsch C. 1998. Abyssal recipes II: Energetics of tidal and wind mixing. Deep-Sea Research 45: 1977-2010</ref>. It has long been recognized that winds and tides are two important sources of mechanical energy to drive the ocean interior mixing. Although most of the tidal energy from Moon and Sun on the global ocean is dissipated in the shallow seas, perhaps 1.0 TW or more of the tidal energy dissipation occurs in the deep ocean through the scattering by ocean-bottom topography of surface tides into internal tidal waves (Egbert and Ray, 2000) <ref>Egbert, G. D., and Ray R. D. 2000. Significant dissipation of tidal energy in the deep ocean inferred from satellite altimeter data. Nature 405: 775-778</ref>. The breaking of internal waves is believed to be a principal contributor to pelagic turbulence. | ||
+ | |||
+ | The winds can also generate internal gravity waves in the surface layer of Earth’s oceans, which are called near-inertial oscillations due to the peak wave energy near the inertial frequency. They are thought to play an important role in diapycnal mixing to sustain the global system of thermohaline circulation. But the exact contribution of wind power to these near-inertial motions and wind’s relative importance compared to tidal forces remain topics of vigorous debate. Estimations on near‐inertial wind power input varied widely from 0.3 to 1.5 TW using numerical models. However, recent calculations on the basis of observations suggest that the wind power is only 0.3–0.6 TW, and the strongest flux of energy occurs between 30° and 60° latitudes during the winter season, when storms are the most prevalent (Liu et al., 2019) <ref>Liu, Y., Z. Jing, and Wu L. 2019. Wind power on oceanic near-inertial oscillations in the global ocean estimated from surface drifters. Geophysical Research Letters 46: 2647-2653</ref>. | ||
+ | |||
+ | In recent decades, biogenic mixing is thought to be another significant contributor to ocean mixing (Katija and Dabiri, 2009) <ref>Katija, K., and Dabiri J. O. 2009. A viscosity-enhanced mechanism for biogenic ocean mixing'. Nature. 460: 624-626</ref>. From small zooplankton to large mammals, swimming animals are capable of carrying bottom water with them as they migrate upward, and that movement indeed creates an inversion that results in ocean mixing. The global power input from this process is estimated in the order of a TW of energy, comparable with levels caused by winds and tides. After all, each day, billions of tiny krill and some jellyfish migrate hundreds of meters from the deep ocean toward the surface where they feed. | ||
+ | |||
+ | ===Importance of deep ocean circulation=== | ||
+ | The deep ocean is a huge storehouse of heat, carbon, oxygen and nutrients. Deep ocean circulation regulates uptake, distribution and release of these elements. The low overturning rate stabilizes our global climate. By carrying oxygen into the deeper layers it supports the largest habitat on earth. | ||
+ | |||
+ | ===Deep ocean circulation and climate change=== | ||
+ | Present theories for explaining the deep ocean circulation predict that global warming will have a negative impact on the deep ocean circulation. Most studies have focused on the northern Atlantic <ref>Broecker, W. S. 2003. Does the trigger for abrupt climate change reside in the ocean or in the atmosphere? Science 300: 1519–1522.</ref>. The formation of dense sinking surface water in the Arctic region will be counteracted by a higher atmospheric temperature and by release of fresh water by ice melting. The feeding of the ''Atlantic Meridional Overturning Circulation'', which drives warm ''Gulf Stream'' waters to the north, will thus be reduced. Besides, the density of the ''North Atlantic Deep Water'' will be lower; therefore the cold return current will flow closer to the ocean surface. It is expected that these factors will cause significant cooling of the West European climate. | ||
+ | |||
+ | Ice melting and resulting fresh water releases in the Antarctic region will hamper the formation of ''Antarctic Bottom Water'' (AABW). Model simulations indicate that this may entail considerable warming of deep waters in the entire Pacific; it may also affect the Atlantic by strengthening the ''Atlantic Meridional Overturning Circulation''. The impact of fresh water releases in the Antarctic region on the global climate and sea-level rise could even be greater than the impact of freshening of the Arctic waters, as discussed in the article [[Thermohaline circulation of the oceans]]. | ||
+ | |||
+ | |||
+ | ==Related articles== | ||
+ | :[[Thermohaline circulation of the oceans]] | ||
+ | :[[Seawater density]] | ||
+ | :[[Open oceans]] | ||
+ | :[[Open ocean habitat]] | ||
+ | :[[Shelf sea exchange with the ocean]] | ||
+ | :[[Ocean and shelf tides]] | ||
+ | :[[Coriolis acceleration]] | ||
+ | :[[Ekman transport]] | ||
+ | :[[Geostrophic flow]] | ||
==References== | ==References== | ||
+ | <references/> | ||
+ | |||
+ | ==See also== | ||
+ | S. Rahmstorf: Thermohaline Ocean Circulation. In: Encyclopedia of Quaternary Sciences, Edited by S. A. Elias. Elsevier, Amsterdam 2006. | ||
+ | |||
+ | Stommel, H.M. 1957. A survey of ocean current theory. Deep-Sea Research 4, 149–184. | ||
+ | |||
+ | https://worldoceanreview.com/en/wor-1/climate-system/great-ocean-currents/ | ||
+ | |||
+ | https://www.britannica.com/science/ocean-current | ||
− | |||
− | {{ | + | {{2Authors |
− | | | + | |AuthorID1=16323 |
− | | | + | |AuthorFullName1=Katrien Töpke |
− | | | + | |AuthorName1=Topke K |
+ | |AuthorID2=39084 | ||
+ | |AuthorFullName2=Dehai Song | ||
+ | |AuthorName2=Song D | ||
+ | }} | ||
− | [[Category: | + | [[Category:Physical coastal and marine processes]] |
− | [[Category: | + | [[Category:Ocean and shelf sea]] |
− | [[Category: | + | [[Category:Hydrodynamics]] |
− | [[Category: | + | [[Category:Climate change, impacts and adaptation]] |
Latest revision as of 18:28, 7 August 2024
This article gives an introduction to the main circulation patterns in the ocean. Ocean circulation patterns are strongly influenced by the Earth's rotation as explained in the articles Ekman transport and Geostrophic flow.
Contents
Introduction
By redistributing heat over the globe, ocean currents have a major impact on the global climate. They cause the relative mildness of the Western European climate, for example. Ocean and atmospheric currents form a coupled dynamic system. Instabilities of this system, the El Nino Southern Oscillation (ENSO) in particular, produce important climate fluctuations. Ocean currents not only distribute heat, but they also play a crucial role in the global ecosystem by storing [math]CO_2[/math] and recycling nutrients.
Currents
There are two main types of ocean currents: currents driven mainly by wind and currents mainly driven by density differences. Density depends on temperature and salinity of the water. Cold and salty water is dense and will sink. Warm and less salty water will float. Although tides are generally a dominant driver of water motion in shallow coastal waters, their relative importance in the oceans is less. It should be noted, however, that tides are mainly generated in the oceans (by the gravitational forces of moon and sun) and are amplified when propagating onto the continental shelf (see the article Ocean and shelf tides).
Wind-driven ocean currents
Global wind field
The large-scale global wind field consists of dominating westerly winds at latitudes between 30 and 60 degrees in the northern and southern hemispheres (the Westerlies) and dominating easterly winds in the tropical/subtropical zone (the Trade winds). This wind field pattern results from the low atmospheric pressure in the tropics (warm ascending air) and high atmospheric pressure in the subtropics (cooled descending air). The near-surface air flow - toward the equator at low latitudes and toward the poles at high latitudes (so-called Hadley cells) - is deflected by earth rotation, hence giving rise to the Westerlies and the Trade winds.
Surface currents
Wind stress generates strong currents (up to several m/s) in the ocean surface layer. The thickness of the surface layer entrained by wind is of the order of 500 meters (about the thickness of the thermocline at low- and mid-latitudes), up to a maximum of 2000 m. Due to earth rotation the main ocean current system consists of large anticyclonic gyres (clockwise rotating in the northern hemisphere and anticlockwise in the southern hemisphere) [1]. There are five major gyres: the North Atlantic, the South Atlantic, the North Pacific, the South Pacific and the Indian Ocean Gyre, see figure 1. The Antarctic Circumpolar Current is situated in the Southern Ocean and constantly circles around Antarctica because there are no land masses to interrupt the currents. It is an eastward-flowing current driven by the dominant western winds at this latitude.

The most famous ocean current, the Gulf Stream, is a vast moving mass of water, transporting an enormous amount of heat from the Caribbean across the ocean to Europe. It passes by the US east coast as a narrow jet, due to the northward increase of the Coriolis acceleration [3] and then spreads out as a meandering current over the ocean while generating a series of meso-scale eddies and whirls. The North Atlantic Gyre is completed by the Canary Current in the Eastern Atlantic that transports relatively cold water south and west. The Kuroshio is a warm boundary current in the north-western Pacific, similar to the Gulf Stream. It is part of the large gyre formed by the California Current and the North Equatorial Current. The North Equatorial Current and South Equatorial Current are driven by the easterly trade winds over the Pacific. The Southern Pacific Gyre is completed by the warm West Australian Current and the cold Peru Current.
Upwelling
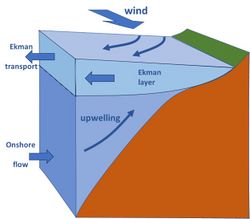
In regions where Ekman transport deflects the boundary current from the coast, water from the deep ocean rises to the ocean surface, see figure 2. This phenomenon is called 'upwelling' and is very important for enrichment of surface waters with organic matter and nutrients. Upwelling zones are characterized by a very rich marine life with abundant resources for fishery. Upwelling zones exist at the southward flowing boundary currents in the Northern Hemisphere (California Current along the US West Coast, Canary Current along the West African coast) and at the northward flowing boundary currents in the Southern Hemisphere (Peru Current along the South American West Coast and Benguela Current along the South African West Coast).
Upwelling also occurs at the equator at the Pacific Ocean (Equatorial upwelling). The North Equatorial Current is deflected to the north and the South Equatorial current to the south as a consequence of the Coriolis effect. This produces upwelling of nutrient rich water and cooling of the surface water near the equator of the Pacific, see figure 3. Downwelling zones exist north and south of the equator, as explained in the article Ekman transport.
El Nino Southern Oscillation
Instability of the coupled ocean-atmosphere dynamics produces large fluctuations in the climate of the Pacific region, which are felt at the global scale. Weakening of the easterly trade winds allows warm water from the Western Pacific to flow back with the Equatorial Counter Current to the eastern South American boundary, where upwelling currents of cold deep ocean water are shut off. This results in relative warming of the Eastern Pacific (lowering the sea surface atmospheric pressure) and relative cooling of the Western Pacific (increasing the sea surface atmospheric pressure) and hence induces a further weakening of the easterly trade winds. This feedback strengthens the so-called El Nino phase of the oscillation [5][6]. The shut-off of the food-rich upwelling currents has major consequences for marine life and fisheries. [7]. After a number of years (three on average, but variable) the system sweeps back to the opposite phase, called La Nina. The onset and offset of the oscillation are still not fully understood.
Deep ocean circulation
Deep ocean circulation is primarily driven by density differences. It is called thermohaline circulation, because density differences are due to temperature and salinity. Density differences are small and the flow velocity is low, of the order of a few cm/s. However, the water masses moving around by thermohaline circulation are huge. Water fluxes are of the order of 20 Sv or more (Sv = Sverdrup = 1 million m3/s). Density gradients alone are not sufficient for sustaining the deep ocean circulation. Upwelling and mixing processes, to bring deep ocean water back to the surface, are required too [8].
Deep water formation
The density of surface water increases when frigid air blows during winter across the ocean at high latitudes. The water density increases further by evaporation and by salt expulsion when sea ice is formed. Deep ocean water masses are formed in the Arctic and Antarctic regions by sinking of dense water with a temperature less than 4°C from the surface to great depth. From these regions, a cold deep water layer spreads over the entire ocean basins.
Conveyor belt
The thermohaline circulation moves water masses around between the different ocean basins [9][10]. 'Ocean conveyor belt' is the popular name of this inter-basin circulation. The conveyor belt is fed in the northern North Atlantic with high-salinity water (due to evaporation) supplied by the Gulf Stream, which sinks to great depth after cooling down in the Arctic region, forming the North Atlantic Deep Water (NADW). The replacement of this dense sinking water generates a continuous surface flow feeding the conveyor belt. The NADW flows from the Arctic region southward, as a deep boundary current along the American shelf [11]. This current compensates for the net northward surface flow in the Atlantic Ocean. This circulation along the north-south axis is called Atlantic Meridional Overturning Circulation (AMOC) , see figure 4.
The NADW finally joins the Antarctic Circumpolar Current and enters the Indian and Pacific oceans. The cold dense water from the Antarctic zone fills the deep water layer in these oceans and then gradually rises and mixes with the surface waters of the Indian and Pacific oceans. The mixing of deep ocean water is promoted by strong surface winds, by tides, by upwelling and by abyssal circulation[12][8]. The circulation is finally completed by a warm surface return current to the Atlantic Ocean that passes south of Africa and America, see figure 5. The whole trip takes more than 1,000 years to complete.

Energy for maintaining the large-scale thermohaline circulation
To maintain the large-scale thermohaline circulation of the ocean, it has been estimated that about 2.1 TW ([math]10^{12}[/math] Watts) of mixing energy is required (Munk and Wunsch, 1998) [14]. It has long been recognized that winds and tides are two important sources of mechanical energy to drive the ocean interior mixing. Although most of the tidal energy from Moon and Sun on the global ocean is dissipated in the shallow seas, perhaps 1.0 TW or more of the tidal energy dissipation occurs in the deep ocean through the scattering by ocean-bottom topography of surface tides into internal tidal waves (Egbert and Ray, 2000) [15]. The breaking of internal waves is believed to be a principal contributor to pelagic turbulence.
The winds can also generate internal gravity waves in the surface layer of Earth’s oceans, which are called near-inertial oscillations due to the peak wave energy near the inertial frequency. They are thought to play an important role in diapycnal mixing to sustain the global system of thermohaline circulation. But the exact contribution of wind power to these near-inertial motions and wind’s relative importance compared to tidal forces remain topics of vigorous debate. Estimations on near‐inertial wind power input varied widely from 0.3 to 1.5 TW using numerical models. However, recent calculations on the basis of observations suggest that the wind power is only 0.3–0.6 TW, and the strongest flux of energy occurs between 30° and 60° latitudes during the winter season, when storms are the most prevalent (Liu et al., 2019) [16].
In recent decades, biogenic mixing is thought to be another significant contributor to ocean mixing (Katija and Dabiri, 2009) [17]. From small zooplankton to large mammals, swimming animals are capable of carrying bottom water with them as they migrate upward, and that movement indeed creates an inversion that results in ocean mixing. The global power input from this process is estimated in the order of a TW of energy, comparable with levels caused by winds and tides. After all, each day, billions of tiny krill and some jellyfish migrate hundreds of meters from the deep ocean toward the surface where they feed.
Importance of deep ocean circulation
The deep ocean is a huge storehouse of heat, carbon, oxygen and nutrients. Deep ocean circulation regulates uptake, distribution and release of these elements. The low overturning rate stabilizes our global climate. By carrying oxygen into the deeper layers it supports the largest habitat on earth.
Deep ocean circulation and climate change
Present theories for explaining the deep ocean circulation predict that global warming will have a negative impact on the deep ocean circulation. Most studies have focused on the northern Atlantic [18]. The formation of dense sinking surface water in the Arctic region will be counteracted by a higher atmospheric temperature and by release of fresh water by ice melting. The feeding of the Atlantic Meridional Overturning Circulation, which drives warm Gulf Stream waters to the north, will thus be reduced. Besides, the density of the North Atlantic Deep Water will be lower; therefore the cold return current will flow closer to the ocean surface. It is expected that these factors will cause significant cooling of the West European climate.
Ice melting and resulting fresh water releases in the Antarctic region will hamper the formation of Antarctic Bottom Water (AABW). Model simulations indicate that this may entail considerable warming of deep waters in the entire Pacific; it may also affect the Atlantic by strengthening the Atlantic Meridional Overturning Circulation. The impact of fresh water releases in the Antarctic region on the global climate and sea-level rise could even be greater than the impact of freshening of the Arctic waters, as discussed in the article Thermohaline circulation of the oceans.
Related articles
- Thermohaline circulation of the oceans
- Seawater density
- Open oceans
- Open ocean habitat
- Shelf sea exchange with the ocean
- Ocean and shelf tides
- Coriolis acceleration
- Ekman transport
- Geostrophic flow
References
- ↑ Munk, W. H. 1950. On the wind-driven ocean circulation. J. Met. 7, 79-93.
- ↑ https://commons.wikimedia.org/wiki/File:Corrientes-oceanicas-en.svg
- ↑ Stommel, H. 1948. The westward intensification of wind-driven ocean currents. Transactions, American Geophysical Union, 29: 202-206.
- ↑ http://www-das.uwyo.edu/~geerts/cwx/notes/chap11/equat_upwel.html
- ↑ Bjerknes, J. 1969. Atmospheric teleconnections from the equatorial Pacific. Mon Weather Rev. 97:163–172.
- ↑ Wyrtki, K. 1973. Teleconnections in the Equatorial Pacific Ocean. Science 180: 66-68.
- ↑ Rice T. 2000. Deep Ocean. The natural history museum, London.
- ↑ 8.0 8.1 Rahmstorf, S. 2006. Thermohaline Ocean Circulation. In: Encyclopedia of Quaternary Sciences, Edited by S. A. Elias. Elsevier.
- ↑ Wüst, G. 1968. History of investigations of the longitudinal deep-sea circulation (1800–1922). Bulletin de l’Institut Oceanographique, Monaco, Numero Special 2, 109–120.
- ↑ Stommel H. and Arons, A.B. 1960. On the abyssal circulation of the world ocean—II. An idealized model of the circulation pattern and amplitude in oceanic basins. Deep-Sea Research 6: 217–233.
- ↑ Stommel H., Arons, A.B. and Faller, A.J. 1958. Some examples of stationary flow patterns in bounded basins. Tellus 10 (2): 179–187.
- ↑ Stommel H. 1958. The abyssal circulation. Deep-Sea Research 5 (1): 80–82.
- ↑ Broecker, W.S. 1991. The Great Ocean conveyor. Oceanography 4: 79–89
- ↑ Munk, W. H., and Wunsch C. 1998. Abyssal recipes II: Energetics of tidal and wind mixing. Deep-Sea Research 45: 1977-2010
- ↑ Egbert, G. D., and Ray R. D. 2000. Significant dissipation of tidal energy in the deep ocean inferred from satellite altimeter data. Nature 405: 775-778
- ↑ Liu, Y., Z. Jing, and Wu L. 2019. Wind power on oceanic near-inertial oscillations in the global ocean estimated from surface drifters. Geophysical Research Letters 46: 2647-2653
- ↑ Katija, K., and Dabiri J. O. 2009. A viscosity-enhanced mechanism for biogenic ocean mixing'. Nature. 460: 624-626
- ↑ Broecker, W. S. 2003. Does the trigger for abrupt climate change reside in the ocean or in the atmosphere? Science 300: 1519–1522.
See also
S. Rahmstorf: Thermohaline Ocean Circulation. In: Encyclopedia of Quaternary Sciences, Edited by S. A. Elias. Elsevier, Amsterdam 2006.
Stommel, H.M. 1957. A survey of ocean current theory. Deep-Sea Research 4, 149–184.
https://worldoceanreview.com/en/wor-1/climate-system/great-ocean-currents/
https://www.britannica.com/science/ocean-current
Please note that others may also have edited the contents of this article.
|