Difference between revisions of "Thermohaline circulation of the oceans"
Dronkers J (talk | contribs) |
|||
(35 intermediate revisions by 5 users not shown) | |||
Line 1: | Line 1: | ||
+ | |||
==Introduction== | ==Introduction== | ||
− | The Thermohaline Circulation (THC) also referred to as the “Great Ocean Conveyor” or the Meridional Overturning Circulation (MOC), can be defined as the density-impelled circulation of the oceans. Thermohaline is derived from the Greek: thermo- for heat and -haline for salt, which constitute the density of water. The water masses transport both energy (heat) and matter (solids, dissolved substances and gasses) around the globe. Changes in the Thermohaline Circulation alter the global ocean heat transport and affect the global climate.(Broecker, W., 1991<ref>Broecker, W., 1991. The great ocean conveyor. Oceanography 1, 79–89.</ref>) | + | The Thermohaline Circulation (THC) also referred to as the “Great Ocean Conveyor” or the Meridional Overturning Circulation (MOC), can be defined as the density-impelled circulation of the oceans. Thermohaline is derived from the Greek: thermo- for heat and -haline for salt, which constitute the [[Seawater density|density of water]]. The water masses transport both energy (heat) and matter (solids, dissolved substances and gasses) around the globe. Changes in the Thermohaline Circulation alter the global ocean heat transport and affect the global climate.(Broecker, W., 1991<ref>Broecker, W., 1991. The great ocean conveyor. Oceanography 1, 79–89.</ref>). See also the article [[Ocean circulation]]. |
− | ==Functioning of the | + | ==Functioning of the Thermohaline Circulation (THC)== |
− | The conveyor belt (Fig.1) has its start near Greenland and Iceland in the North Atlantic, where seawater at the surface of the ocean is intensively cooled by means of a wind-driven process called evaporative cooling. | + | The conveyor belt (Fig.1) has its start near Greenland and Iceland in the North Atlantic, where seawater at the surface of the ocean is intensively cooled by means of a wind-driven process called evaporative cooling. Only the pure water molecules are removed during evaporation, resulting in an increase in the salinity of the seawater and therefore an increase in the [[Seawater density|density]] of the water mass. Evaporative cooling is predominant in the vicinity of the Norwegian Sea, and the sinking water mass known as the North Atlantic Deep Water (NADW), fills the basin and moves southwards through the crevasses in the submarine sills that connect Greenland, Iceland and Great Britain. From there, it flows very slowly into the deep [[abyssal plain| abyssal plains]] of the Atlantic toward Antarctica where the water mass joins the Antarctic Circumpolar Current. Flow from the Arctic Ocean Basin into the Pacific is blocked by the narrow shallows of the Bering Strait. |
+ | [[Image:Thermohaline.jpg|thumb|right|500px|Fig.1. The Thermohaline Circulation. Source: IPPC 2001.]] | ||
− | |||
+ | In the Weddell Sea (Antarctica), the combined effect of evaporative cooling and brine exclusion cause the [[Seawater density|density]] of the Antarctic Bottom Water (AABW) to be so high, that it in fact underflows the NADW in the Atlantic Basin. Flow of the AABW into the Pacific is blocked by the Drake Passage between the Antarctic Peninsula and the southernmost tip of South America. Within the Antarctic Circumpolar Current, the AABW and NADW are mixed to create the so-called “Common Water” which flows northward, around Southern Africa where it is split into two routes: one into the Indian Ocean and the other past Australia into the Pacific. In the Indian Ocean, some of the cold and salty water from the Atlantic are refreshed, by means of vertical exchange, with the warmer upper ocean water from the tropical Pacific. Within the Pacific Ocean, the remaining cold water undergoes Haline forcing (net high latitude freshwater gain and low latitude evaporation) and slowly becomes warmer and fresher. The outflow of bottom water makes the sea level of the Atlantic slightly lower than the Pacific. Combined with the difference in salinity, this generates a large flow of warmer upper ocean water from the tropical Pacific to the Indian Ocean through the Indonesian Archipelago to replace the AABW. From there, the water flows up through the South Atlantic towards the tropics. The heated tropical waters of the Atlantic proceed until Greenland, where it once more undergoes evaporative cooling, thereby creating a continuous global thermohaline circulation. | ||
+ | ==Bi-polar characteristic of the Thermohaline Circulation== | ||
− | + | Traditionally, research has been almost exclusively devoted to understanding the sensitivity of the high-latitudinal oceans (especially in the Northern Hemisphere) to freshwater fluxes. These studies have advanced considerably the comprehension of the dynamics and functioning of the North Atlantic Bottom Water (NABW) circulation. In comparison therewith, little is known about the southern sources of deepwater (AABW). It is important to bear in mind that the thermohaline circulation is operated by both deepwater sources, and therefore, the deficit of scientific knowledge limits the complete understanding of decadal to millennial time-scale climate change (Seidov, D., 2000<ref name="seidov">Seidov, D., Barron, E., Haupt, B. J., 2000. Meltwater and the global ocean conveyor: northern versus southern connections. Global and Planetary Change 30, 257-270.</ref>). An example of such a deficit is whether the NADW is the ultimate driver of the conveyor, and if additional variability is generated by freshwater impacts in the Southern Ocean (Seidov, D., 2000<ref name="seidov"/>). A significant influence of the Southern Ocean is supported by several scientific lines of evidence. First, many examples of climate intermittency during the glacial cycles of the Pleistocene remain poorly understood, even though they seem to correlate with major deglaciations(Seidov, D., 2000<ref name="seidov"/>). Second, recent studies (Blunier, T. et al. 1998<ref>, 1998. Asynchrony of Antarctic and Greenland climate change during the last glacial period. Nature 394, 739–743.</ref>, Broecker, W.S., 1994<ref>Broecker, W.S., 1994. Massive iceberg discharges as triggers for global climate change. Nature 372, 421–424.</ref>, Stocker, T.F., 1998 <ref>Stocker, T.F., 1998. The seesaw effect. Science 282, 61–62.</ref>) reveal a bi-polar nature of the glacial cycles of the Pleistocene, e.g. the southern Atlantic leads in the occurrence of several Heinrich Events (Vidal, L. et al., 1999<ref>Vidal, L. et al., 1999. Link between the North and South Atlantic during the [[Heinrich events]] of the last glacial period. Clim. Dyn. 15, 909–919.</ref>). Another study (Birchfield, G.E., Broekcer, W.S., 1990<ref name="birchfield">Birchfield, G.E., Broecker, W.S., 1990. A salt oscillator in the glacial Atlantic? 2. A scale analysis model. Paleoceanography 5, 835–843.</ref>) argues that the Little Ice Age (circa 500 years ago) was caused by far stronger deep ocean ventilation in the Southern Ocean. One reason put forward for enhanced southern ocean ventilation is an increase in Atlantic Ocean salinity. Conversely, a slowdown in ventilation could be caused by reduced surface salinity, associated warming, and sea ice or ice sheet melting in the Southern Ocean after the Little Ice Age(Seidov, D., 2000<ref name="seidov"/>, Broecker, W.S., 2000<ref>Broecker, W.S., 2000. Was a change in thermohaline circulation responsible for the Little Ice Age? Proc. Natl. Acad. Sci. 97 (4), 1339–1342.</ref>). The potential importance of feedbacks between the northern and southern sources of deepwater is still largely unknown. | |
− | |||
− | |||
− | |||
− | Traditionally, research has been almost exclusively devoted to understanding the sensitivity of the high-latitudinal oceans (especially in the Northern Hemisphere) to freshwater fluxes. These studies have advanced considerably the comprehension of the dynamics and functioning of the North Atlantic Bottom Water (NABW) circulation. In comparison therewith, little is known about the southern sources of deepwater (AABW). It is important to bear in mind that the thermohaline circulation is operated by both deepwater sources, and therefore, the deficit of scientific knowledge limits the complete understanding of decadal to millennial time-scale climate change (Seidov, D., 2000<ref name="seidov">Seidov, D., Barron, E., Haupt, B. J., 2000. Meltwater and the global ocean conveyor: northern versus southern connections. Global and Planetary Change 30, 257-270.</ref>). An example of such a deficit is whether the NADW is the ultimate driver of the conveyor, and if additional variability is generated by freshwater impacts in the Southern Ocean (Seidov, D., 2000<ref name="seidov"/>). A significant influence of the Southern Ocean is supported by several scientific lines of evidence. First, many examples of climate intermittency during the glacial cycles of the Pleistocene remain poorly understood, even though they seem to correlate with major deglaciations(Seidov, D., 2000<ref name="seidov"/>). Second, recent studies (Blunier, T. et al. 1998<ref>, 1998. Asynchrony of Antarctic and Greenland climate change during the last glacial period. Nature 394, 739–743.</ref>, Broecker, W.S., 1994<ref>Broecker, W.S., 1994. Massive iceberg discharges as triggers for global climate change. Nature 372, 421–424.</ref>, Stocker, T.F., 1998 <ref>Stocker, T.F., 1998. The seesaw effect. Science 282, 61–62.</ref>) reveal a bi-polar nature of the glacial cycles of the Pleistocene, e.g. the southern Atlantic leads in the occurrence of several Heinrich Events (Vidal, L. et al., 1999<ref>Vidal, L. et al., 1999. Link between the North and South Atlantic during the Heinrich events of the last glacial period. Clim. Dyn. 15, 909–919.</ref>). Another study (Birchfield, G.E., Broekcer, W.S., 1990<ref name="birchfield">Birchfield, G.E., Broecker, W.S., 1990. A salt oscillator in the glacial Atlantic? 2. A scale analysis model. Paleoceanography 5, 835–843.</ref>) argues that the Little Ice Age (circa 500 years ago) was caused by far stronger deep ocean ventilation in the Southern Ocean. One reason put forward for enhanced southern ocean ventilation is an increase in Atlantic Ocean salinity. Conversely, a slowdown in ventilation could be caused by reduced surface salinity, associated warming, and sea ice or ice sheet melting in the Southern Ocean after the Little Ice Age(Seidov, D., 2000<ref name="seidov"/>, Broecker, W.S., 2000<ref>Broecker, W.S., 2000. Was a change in thermohaline circulation responsible for the Little Ice Age? Proc. Natl. Acad. Sci. 97 (4), 1339–1342.</ref>). The potential importance of feedbacks between the northern and southern sources of deepwater is still largely unknown. | ||
==Freshwater sources in the Southern Ocean== | ==Freshwater sources in the Southern Ocean== | ||
Line 26: | Line 25: | ||
The ocean, including its abyss, is warming at a rate of 0.5˚C or more per century (Levitus, S., 2000<ref>Levitus, S., Antonov, J.I., Boyer, T.P., Stephens, C., 2000. Warming of the world ocean. Science 287, 2225–2229.</ref>). Although historical observations and paleoclimatic data reveal significant climate variability on decadal to millennial time scales, this ocean warming during the last several decades is linked to global climate change. Changes in the atmospheric abundance of greenhouse gases and aerosols, in solar radiation and land surface properties have altered the energy balance of the climate system. | The ocean, including its abyss, is warming at a rate of 0.5˚C or more per century (Levitus, S., 2000<ref>Levitus, S., Antonov, J.I., Boyer, T.P., Stephens, C., 2000. Warming of the world ocean. Science 287, 2225–2229.</ref>). Although historical observations and paleoclimatic data reveal significant climate variability on decadal to millennial time scales, this ocean warming during the last several decades is linked to global climate change. Changes in the atmospheric abundance of greenhouse gases and aerosols, in solar radiation and land surface properties have altered the energy balance of the climate system. | ||
− | The global atmospheric concentration of carbon dioxide has increased from a pre-industrial level of 280 ppm to 379 ppm in 2005(Geo Year Book, 2004/2005<ref name="year">Geo Year Book 2004/2005: An overview of our changing environment. United Nations Environment Program. 80-84.</ref>). This atmospheric concentration of carbon dioxide exceeds by far the natural range over the last 650,000 years (180 to 300 ppm)(Geo Year Book, 2004/2005<ref name="year"/>) as determined from ice cores. Consequently, the change of the global energy balance has seen a decrease in sea ice (Fig. 2) as well as rapid ice sheet and permafrost melting. This excess of high latitudinal freshwater influx can substantially modify the deep ocean circulation. | + | The global atmospheric concentration of carbon dioxide has increased from a pre-industrial level of 280 parts per million (ppm) to 379 ppm in 2005 (Geo Year Book, 2004/2005<ref name="year">Geo Year Book 2004/2005: An overview of our changing environment. United Nations Environment Program. 80-84.</ref>). This atmospheric concentration of carbon dioxide exceeds by far the natural range over the last 650,000 years (180 to 300 ppm) (Geo Year Book, 2004/2005<ref name="year"/>) as determined from ice cores. Consequently, the change of the global energy balance has seen a decrease in sea ice (Fig. 2) as well as rapid ice sheet and permafrost melting. This excess of high latitudinal freshwater influx can substantially modify the deep ocean circulation. |
− | Fig. 2. Melting sea ice | + | [[Image:Melting ice sea.jpg|thumb|right|300px|Fig. 2. Melting sea ice]] |
− | The sinking that drives the thermohaline circulation depends critically on the water being sufficiently cold and salty. Therefore, any factor that changes the state of the conditions for circulation, can result in a slow-down of the thermohaline, and thereby dramatically influence the climatic state and driving further climate change. Observations over recent decades suggest that changes in the factors governing circulation are already occurring (Geo Year Book, 2004/2005<ref name="year"/>). This raises concerns about potential abrupt climate changes in the future. Is a complete shutdown of the thermohaline possible? | + | The sinking that drives the thermohaline circulation depends critically on the water being sufficiently cold and salty. Therefore, any factor that changes the state of the conditions for circulation, can result in a slow-down of the thermohaline circulation, and thereby dramatically influence the climatic state and driving further [[climate change]]. Observations over recent decades suggest that changes in the factors governing circulation are already occurring (Geo Year Book, 2004/2005<ref name="year"/>). This raises concerns about potential abrupt climate changes in the future. Is a complete shutdown of the thermohaline possible? |
===High Latitudinal Freshwater Influx=== | ===High Latitudinal Freshwater Influx=== | ||
Line 45: | Line 44: | ||
===Sea-Level Change=== | ===Sea-Level Change=== | ||
− | |||
− | Fig.3. IPCC summary of the observed variations in the cryosphere for the years 1993-2003. | + | [[Image:Figure_3.jpg|thumb|left|500px|Fig.3. IPCC summary of the observed variations in the cryosphere for the years 1993-2003 <ref>Lemke, P., J. Ren, R.B. Alley, I. Allison, J. Carrasco, G. Flato, Y. Fujii, G. Kaser, P. Mote, R.H. Thomas and T. Zhang, 2007: Observations: Changes in Snow, Ice and Frozen Ground. In: Climate Change 2007: The Physical Science Basis. Contribution of Working Group I to the Fourth Assessment Report of the Intergovernmental Panel on Climate Change [Solomon, S., D. Qin, M. Manning, Z. Chen, M. Marquis, K.B. Averyt, M. Tignor and H.L. Miller (eds.)]. Cambridge University Press, Cambridge, United Kingdom and New York, NY, US, Pp. 375 </ref>]] |
− | Fig. 4: Differences of the sea-level elevations in cm relative to the current situation and | + | [[Sea level rise]] can be caused by either melting of major ice sheets (Fig. 3), or as an indirect effect by freshwater influx events caused by thermal restructuring of the world ocean. As the deep ocean warms up, the sea elevation will change as a result of the thermal expansion of sea water. Historic [http://en.wikipedia.org/wiki/Hydrography hydrographic] data suggest that thermal expansion of the ocean can contribute tens of centimeters to the observed [[sea level rise]] over the last century (Godfrey, J.S., Love, G., 1992<ref>Godfrey, J.S., Love, G., 1992. Assessment of sealevel rise, specific to the South Asian and Australian situations. Seal Level Changes: Determination and Effects. Geophys. Monogr., vol. 69. AGU, Washington, DC, pp. 87–94. </ref>). Some simulations (Church, J.A., Godfrey, J.S., Jacket, D.R., McDougall, T.R., 1991<ref>Church, J.A., Godfrey, J.S., Jacket, D.R., McDougall, T.R., 1991. A model of sealevel rise caused by ocean thermal expansion. J. Clim. 4, 438–456.</ref> indicate that the thermal expansion of the ocean associated with a global warming of 3˚C temperature rise by the year 2050 results in up to 30 cm sea-level rise. In the simulation experiment conducted, (Seidov, D., 2000<ref name="seidov"/>) a significant sea-level rise of approximately 2-3 meter may occur during a Southern Ocean event (Fig. 4). In many sensitive coastal areas the sea-level rise could be over 1 meter. It is important to note that this sea-level rise could occur without significant melting of the ice sheets, including the Western Antarctic Ice Sheet (WAIS), which is considered the most vulnerable to climate change(Seidov, D., 2000<ref name="seidov"/>). |
− | a freshwater influx event in the North Atlantic and the Southern Ocean(Seidov, D., 2000<ref name="seidov"/>) | + | |
+ | |||
+ | [[Image:Differences.jpg|thumb|center|900px|Fig. 4: Differences of the sea-level elevations in cm relative to the current situation and a freshwater influx event in the North Atlantic a) and the Southern Ocean b) (Seidov, D., 2000<ref name="seidov"/>)]] | ||
===Indirect Impacts Resulting from Changes in the Thermohaline Circulation=== | ===Indirect Impacts Resulting from Changes in the Thermohaline Circulation=== | ||
− | The changing climatic conditions and the increased freshwater influx in the Polar Regions have seen sea ice retreats from the coastline of Arctic countries by between | + | The changing climatic conditions and the increased freshwater influx in the Polar Regions have seen sea ice retreats from the coastline of Arctic countries by between 150 km and 200 km. According to the [http://www.ipcc.ch/ International Panel on Climate Change] (IPCC), by 2050 the sea ice might retreat up to 800 km as a result of global warming. The loss of ice in the Polar Regions could lead to a sudden acceleration of global warming, as ice reflects radiation or heat from the sun back into space. The absence of sea ice combined with deep ocean warming will see more evaporation and rainfall occurring in these sensitive regions, which in turn will speed up sea ice loss. |
The retreat and loss of sea ice in the Polar Regions will also have a harmful impact on the many species living in the regions. Polar bears, for example, need ice so that they can hunt for seals. A loss of sea ice could make it harder for these animals to acquire enough food. Pregnant females and those with cubs may be particularly at risk. The species of seal that need ice for resting or pup rearing will also be at risk. | The retreat and loss of sea ice in the Polar Regions will also have a harmful impact on the many species living in the regions. Polar bears, for example, need ice so that they can hunt for seals. A loss of sea ice could make it harder for these animals to acquire enough food. Pregnant females and those with cubs may be particularly at risk. The species of seal that need ice for resting or pup rearing will also be at risk. | ||
− | As mentioned above, the change of the thermohaline circulation is expected to alter the speed and patterns of ocean currents, which in turn will impact fish stocks. According to the Third Assessment Report of the IPCC: “Arctic fisheries are among the most productive in the world. Changes in the velocity and direction of ocean currents affect the availability of nutrients and the disposition of larval and juvenile organisms, thereby influencing recruitment, growth and mortality.” Changes in the fish stocks are already taking place, with the result that some stocks are boosting while others are damaged. For example, Groundfish stocks have shown a positive response to recent climate change, while the thermally sensitive Greenland turbot and King crab stocks in the eastern Bering Sea and Kodiak are declining. The IPCC | + | As mentioned above, the change of the thermohaline circulation is expected to alter the speed and patterns of [[ocean currents]], which in turn will impact [[fish stocks]]. According to the [http://www.ipcc.ch/ipccreports/assessments-reports.htm Third Assessment Report] of the [http://www.ipcc.ch/ IPCC]: “Arctic fisheries are among the most productive in the world. Changes in the velocity and direction of ocean currents affect the availability of [[nutrients]] and the disposition of larval and juvenile organisms, thereby influencing recruitment, growth and mortality.” Changes in the fish stocks are already taking place, with the result that some stocks are boosting while others are damaged. For example, Groundfish stocks have shown a positive response to recent climate change, while the thermally sensitive Greenland turbot and King crab stocks in the eastern Bering Sea and Kodiak are declining. The IPCC anticipates that harvests might halve or double depending on the stocks concerned; meanwhile some fisheries might die out altogether while new ones develop. This could increase or decrease local economies by hundreds of millions of dollars annually (IPCC). These projected changes in the [[abundance]] and distribution of fish stocks and wildlife are just one of the issues facing fishermen, Arctic communities and the indigenous people. |
− | The Polar Regions annually | + | The Polar Regions annually accumulate pollution, including [[Portal:Ecotox|Persistent Organic Pollutants]] (POPs), which have been discharged from industry and agriculture around the globe. Melting of the sea ice could trigger the release of these pollutants which could cause them to re-enter the food chain. This would pose a health threat to top predators, e.g. polar bears and humans in these regions. |
On the other hand, the melting of sea ice could have one economic benefit, namely in the area of shipping. The northwards retreat of sea ice opens up the Northern Sea Route, which would allow vessels to sail from Europe to the Far East by going north of Russia rather than using the existing route, i.e. the Suez Canal. Although this should reduce sailing times, cutting the costs of goods and air pollution, the risk of accidents leading to oil spills still remains. | On the other hand, the melting of sea ice could have one economic benefit, namely in the area of shipping. The northwards retreat of sea ice opens up the Northern Sea Route, which would allow vessels to sail from Europe to the Far East by going north of Russia rather than using the existing route, i.e. the Suez Canal. Although this should reduce sailing times, cutting the costs of goods and air pollution, the risk of accidents leading to oil spills still remains. | ||
Line 67: | Line 67: | ||
The major impact for coastal regions caused by a change in the thermohaline circulation will be rising sea levels. There are many coastal sensitive regions around the globe which will be affected by even a small change in the sea level. As indicated above, the changing circulation can rise sea levels by either: melting of sea ice and ice sheets in the Polar Regions or via thermal expansion of the sea water. In 2007 approximately 634 million people lived in coastal areas within 9.1m of the sea level. Two thirds of the world’s cities with more than five million people are located in these low-lying coastal areas. | The major impact for coastal regions caused by a change in the thermohaline circulation will be rising sea levels. There are many coastal sensitive regions around the globe which will be affected by even a small change in the sea level. As indicated above, the changing circulation can rise sea levels by either: melting of sea ice and ice sheets in the Polar Regions or via thermal expansion of the sea water. In 2007 approximately 634 million people lived in coastal areas within 9.1m of the sea level. Two thirds of the world’s cities with more than five million people are located in these low-lying coastal areas. | ||
− | The IPCC Working Group II report indicates that current and future climate change would be expected to have a number of impacts, especially on coastal systems. Potential impacts may include: increased coastal erosion, higher storm | + | The IPCC Working Group II report indicates that current and future climate change would be expected to have a number of impacts, especially on coastal systems. Potential impacts may include: increased [[Natural causes of coastal erosion|coastal erosion]], higher [[Extreme storms|storm surge]] flooding, inhibition of [[primary production]] processes, more extensive coastal inundation, changes in surface water quality and groundwater characteristics, increased loss of property and coastal habitats, increased [[Decision Support Systems for coastal risk assessment and management|flood risk]] and potential loss of life, loss of non-monetary cultural resources and values, impacts on agriculture and aquaculture via a decline in soil and water quality, and loss of tourism, recreation and transportation functions. Furthermore the IPCC report concludes that due to the great diversity of coastal environments; regional and local differences in projected relative sea level and climate changes; and differences in the [[resilience]] and [[adaptive capacity]] of [[ecosystems]], sectors and countries, the impacts will be highly variable in time and space and will not necessarily be negative in all situations. |
==See also== | ==See also== | ||
− | === | + | ===Related articles=== |
− | + | :[[Ocean circulation]] | |
+ | :[[Seawater density]] | ||
+ | :[[Sea level rise]] | ||
+ | :[[Shelf sea exchange with the ocean]] | ||
===External Links=== | ===External Links=== | ||
Line 79: | Line 82: | ||
* IPPC: http://www.ipcc.ch/ | * IPPC: http://www.ipcc.ch/ | ||
* UNEP: http://unep.org | * UNEP: http://unep.org | ||
− | + | ||
==References== | ==References== | ||
<references/> | <references/> | ||
− | |||
{{author | {{author | ||
Line 89: | Line 91: | ||
|AuthorFullName=Tange, Hannli | |AuthorFullName=Tange, Hannli | ||
|AuthorName=Tange, Hannli}} | |AuthorName=Tange, Hannli}} | ||
− | [[Category: | + | |
− | [[Category: | + | |
− | [[Category: | + | [[Category:Physical coastal and marine processes]] |
+ | [[Category:Ocean and shelf sea]] | ||
+ | [[Category:Hydrodynamics]] | ||
+ | [[Category:Climate change, impacts and adaptation]] | ||
+ | [[Category:Sea level rise]] | ||
+ | [[Category:Coastal and marine ecosystems]] |
Latest revision as of 16:38, 22 December 2020
Contents
Introduction
The Thermohaline Circulation (THC) also referred to as the “Great Ocean Conveyor” or the Meridional Overturning Circulation (MOC), can be defined as the density-impelled circulation of the oceans. Thermohaline is derived from the Greek: thermo- for heat and -haline for salt, which constitute the density of water. The water masses transport both energy (heat) and matter (solids, dissolved substances and gasses) around the globe. Changes in the Thermohaline Circulation alter the global ocean heat transport and affect the global climate.(Broecker, W., 1991[1]). See also the article Ocean circulation.
Functioning of the Thermohaline Circulation (THC)
The conveyor belt (Fig.1) has its start near Greenland and Iceland in the North Atlantic, where seawater at the surface of the ocean is intensively cooled by means of a wind-driven process called evaporative cooling. Only the pure water molecules are removed during evaporation, resulting in an increase in the salinity of the seawater and therefore an increase in the density of the water mass. Evaporative cooling is predominant in the vicinity of the Norwegian Sea, and the sinking water mass known as the North Atlantic Deep Water (NADW), fills the basin and moves southwards through the crevasses in the submarine sills that connect Greenland, Iceland and Great Britain. From there, it flows very slowly into the deep abyssal plains of the Atlantic toward Antarctica where the water mass joins the Antarctic Circumpolar Current. Flow from the Arctic Ocean Basin into the Pacific is blocked by the narrow shallows of the Bering Strait.
In the Weddell Sea (Antarctica), the combined effect of evaporative cooling and brine exclusion cause the density of the Antarctic Bottom Water (AABW) to be so high, that it in fact underflows the NADW in the Atlantic Basin. Flow of the AABW into the Pacific is blocked by the Drake Passage between the Antarctic Peninsula and the southernmost tip of South America. Within the Antarctic Circumpolar Current, the AABW and NADW are mixed to create the so-called “Common Water” which flows northward, around Southern Africa where it is split into two routes: one into the Indian Ocean and the other past Australia into the Pacific. In the Indian Ocean, some of the cold and salty water from the Atlantic are refreshed, by means of vertical exchange, with the warmer upper ocean water from the tropical Pacific. Within the Pacific Ocean, the remaining cold water undergoes Haline forcing (net high latitude freshwater gain and low latitude evaporation) and slowly becomes warmer and fresher. The outflow of bottom water makes the sea level of the Atlantic slightly lower than the Pacific. Combined with the difference in salinity, this generates a large flow of warmer upper ocean water from the tropical Pacific to the Indian Ocean through the Indonesian Archipelago to replace the AABW. From there, the water flows up through the South Atlantic towards the tropics. The heated tropical waters of the Atlantic proceed until Greenland, where it once more undergoes evaporative cooling, thereby creating a continuous global thermohaline circulation.
Bi-polar characteristic of the Thermohaline Circulation
Traditionally, research has been almost exclusively devoted to understanding the sensitivity of the high-latitudinal oceans (especially in the Northern Hemisphere) to freshwater fluxes. These studies have advanced considerably the comprehension of the dynamics and functioning of the North Atlantic Bottom Water (NABW) circulation. In comparison therewith, little is known about the southern sources of deepwater (AABW). It is important to bear in mind that the thermohaline circulation is operated by both deepwater sources, and therefore, the deficit of scientific knowledge limits the complete understanding of decadal to millennial time-scale climate change (Seidov, D., 2000[2]). An example of such a deficit is whether the NADW is the ultimate driver of the conveyor, and if additional variability is generated by freshwater impacts in the Southern Ocean (Seidov, D., 2000[2]). A significant influence of the Southern Ocean is supported by several scientific lines of evidence. First, many examples of climate intermittency during the glacial cycles of the Pleistocene remain poorly understood, even though they seem to correlate with major deglaciations(Seidov, D., 2000[2]). Second, recent studies (Blunier, T. et al. 1998[3], Broecker, W.S., 1994[4], Stocker, T.F., 1998 [5]) reveal a bi-polar nature of the glacial cycles of the Pleistocene, e.g. the southern Atlantic leads in the occurrence of several Heinrich Events (Vidal, L. et al., 1999[6]). Another study (Birchfield, G.E., Broekcer, W.S., 1990[7]) argues that the Little Ice Age (circa 500 years ago) was caused by far stronger deep ocean ventilation in the Southern Ocean. One reason put forward for enhanced southern ocean ventilation is an increase in Atlantic Ocean salinity. Conversely, a slowdown in ventilation could be caused by reduced surface salinity, associated warming, and sea ice or ice sheet melting in the Southern Ocean after the Little Ice Age(Seidov, D., 2000[2], Broecker, W.S., 2000[8]). The potential importance of feedbacks between the northern and southern sources of deepwater is still largely unknown.
Freshwater sources in the Southern Ocean
The source and the character of the freshwater sources in the Southern Hemisphere are different from the Northern Hemisphere. Sea surface salinity (SSS) controls both the Antarctic Bottom Water and Antarctic Intermediate Water northward incursions. The SSS can either be increased due to brine exclusion (during the formation of sea ice) or decreased due to sea ice melting. Sea ice formation and brine exclusion rates therefore play a vital role in the southern circulation regime. Additionally, the Antarctic ice sheet has an important function in governing freshwater fluxes into the Southern Ocean. Concern however has been raised about the stability of the West Antarctic Ice Sheet (WAIS). The Antarctic ice sheet mass balance and its possible contribution to global sea level rise is a major issue of debate, since the potential for changes in freshwater fluxes or salinity variations to influence the Southern Ocean is clearly evident. It has been indicated (Birchfield, G.E., Broekcer, W.S., 1990[7]) that even a relatively small freshwater influx converted to a low-salinity signal will hamper the effectiveness of the conveyor operation. These factors have initiated a growing modelling effort designed to investigate the climatic role of the Southern Hemisphere (Seidov, D., 2000[2]) .
The role of global climate change
The ocean, including its abyss, is warming at a rate of 0.5˚C or more per century (Levitus, S., 2000[9]). Although historical observations and paleoclimatic data reveal significant climate variability on decadal to millennial time scales, this ocean warming during the last several decades is linked to global climate change. Changes in the atmospheric abundance of greenhouse gases and aerosols, in solar radiation and land surface properties have altered the energy balance of the climate system.
The global atmospheric concentration of carbon dioxide has increased from a pre-industrial level of 280 parts per million (ppm) to 379 ppm in 2005 (Geo Year Book, 2004/2005[10]). This atmospheric concentration of carbon dioxide exceeds by far the natural range over the last 650,000 years (180 to 300 ppm) (Geo Year Book, 2004/2005[10]) as determined from ice cores. Consequently, the change of the global energy balance has seen a decrease in sea ice (Fig. 2) as well as rapid ice sheet and permafrost melting. This excess of high latitudinal freshwater influx can substantially modify the deep ocean circulation.
The sinking that drives the thermohaline circulation depends critically on the water being sufficiently cold and salty. Therefore, any factor that changes the state of the conditions for circulation, can result in a slow-down of the thermohaline circulation, and thereby dramatically influence the climatic state and driving further climate change. Observations over recent decades suggest that changes in the factors governing circulation are already occurring (Geo Year Book, 2004/2005[10]). This raises concerns about potential abrupt climate changes in the future. Is a complete shutdown of the thermohaline possible?
High Latitudinal Freshwater Influx
In light of the deficit of the scientific understanding of the thermohaline circulation and the feedback potentials between the two deepwater sources, it is difficult to predict the influence of global climate change on the dynamics of the thermohaline. Even within the scientific realm there is disagreement on the possibility of a complete shutdown of the thermohaline circulation. Given this discrepancy, a simulation experiment was conducted (Seidov, D., 2000[2]) to analyse the sensitivity of thermohaline circulation to low-salinity perturbations. In this simulation, the authors set out to demonstrate the degree to which the thermohaline circulation is driven by both the NADW and the AABW, by means of a designed series of simplified freshwater influx events, in which all ocean model parameters are held constant except salinity. The results of the experiments were described for: a North Atlantic freshwater influx, a Southern Ocean freshwater influx, and a combined influx for the North Atlantic and Southern Ocean.
- The North Atlantic Freshwater Influx
The results of the experiment simulating a low-salinity impact (-2 psu than present) on the North-Atlantic conform to what is already known from previous work, namely that the conveyor is weaker and shallower. Temperature differences between the current conditions and this low-salinity scenario indicate cooling in high latitudes of the Atlantic Ocean. This occurs because the reduced NADW production led to a shallow conveyor and cooler and fresher water than today in these latitudes characterises the deep ocean water. In addition to this, the surface ocean has more time to lose heat to the atmosphere because the overturning slowed. The reduced NADW outflow has an evident imprint in the oceanic heat transport. Northward cross-equatorial heat transport is dramatically reduced in the scenario with a strong freshwater impact, which indicates the possibility of a cold episode following a freshwater influx event. If the present-day global warming was potent enough to induce a low-salinity episode in the North Atlantic, caused by iceberg and Arctic sea ice melting, the result could be a tendency towards colder temperature conditions in the Northern Hemisphere. It is important though to note that even with an excessive northern low-salinity signal of -2 psu during the simulation, no complete termination of the conveyor occurred.
- The Southern Ocean Freshwater Influx
In contrast to the predictable results of the northern low-salinity impact, the results of the Southern Ocean surface freshening are less intuitive. Two aspects are noteworthy: first the circulation changes driven by the low-salinity signal were much stronger, and second, they led to a very strong warming of the deep ocean. Warming takes place over the entire deep ocean and its maximum shifts to the southern edges. This deep-sea warming is caused not only by a substantial increase (by 40-60%) in NADW production, but also because the meridional overturning takes over the entire deep ocean, pushing away the lessened AABW. In the North Atlantic scenario, the freshwater influx impact on the conveyor caused thermal effects only in the deep Atlantic Ocean, whereas in the Southern Ocean, the freshwater scenarios impact is global. The increased NADW outflow in the deep layers leads to increased compensating northward surface water flow. This flow carries more warm and salty subtropical water to convection sites, which might further increase NADW production until the atmosphere warms up to reduce the cooling of the sea surface and subsequently reduce the deep convection. The positive feedback of NADW production and northward heat transport can be viewed as a first link toward high-latitudinal warming in the Northern Hemisphere caused by freshwater influx events in the Southern Ocean.
- Combined Influx for the North Atlantic and Southern Ocean
The results of a combined low-salinity event of the North Atlantic and the Southern Ocean, display a deepwater regime that is qualitatively similar to a Southern-Ocean-only experiment. Less Deep Ocean warming is observed, however the impact still remains global and substantial. Results of the runs with perturbations to two sources, demonstrates a more powerful response to a freshwater influx event in the Southern Ocean than for those in the North Atlantic. However, much of this power stems from increased NADW production.
Sea-Level Change
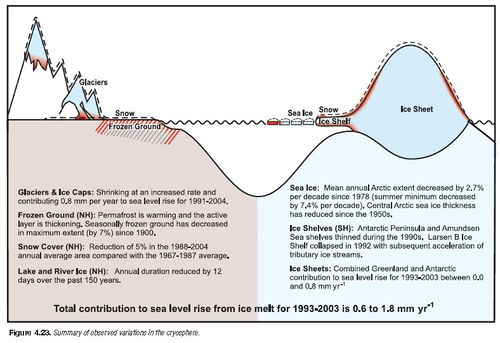
Sea level rise can be caused by either melting of major ice sheets (Fig. 3), or as an indirect effect by freshwater influx events caused by thermal restructuring of the world ocean. As the deep ocean warms up, the sea elevation will change as a result of the thermal expansion of sea water. Historic hydrographic data suggest that thermal expansion of the ocean can contribute tens of centimeters to the observed sea level rise over the last century (Godfrey, J.S., Love, G., 1992[12]). Some simulations (Church, J.A., Godfrey, J.S., Jacket, D.R., McDougall, T.R., 1991[13] indicate that the thermal expansion of the ocean associated with a global warming of 3˚C temperature rise by the year 2050 results in up to 30 cm sea-level rise. In the simulation experiment conducted, (Seidov, D., 2000[2]) a significant sea-level rise of approximately 2-3 meter may occur during a Southern Ocean event (Fig. 4). In many sensitive coastal areas the sea-level rise could be over 1 meter. It is important to note that this sea-level rise could occur without significant melting of the ice sheets, including the Western Antarctic Ice Sheet (WAIS), which is considered the most vulnerable to climate change(Seidov, D., 2000[2]).
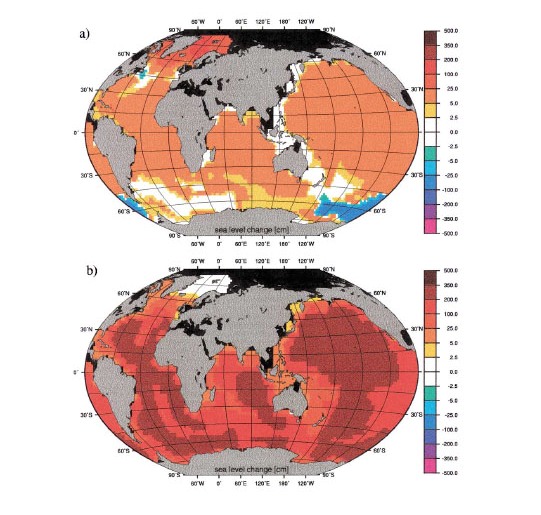
Indirect Impacts Resulting from Changes in the Thermohaline Circulation
The changing climatic conditions and the increased freshwater influx in the Polar Regions have seen sea ice retreats from the coastline of Arctic countries by between 150 km and 200 km. According to the International Panel on Climate Change (IPCC), by 2050 the sea ice might retreat up to 800 km as a result of global warming. The loss of ice in the Polar Regions could lead to a sudden acceleration of global warming, as ice reflects radiation or heat from the sun back into space. The absence of sea ice combined with deep ocean warming will see more evaporation and rainfall occurring in these sensitive regions, which in turn will speed up sea ice loss.
The retreat and loss of sea ice in the Polar Regions will also have a harmful impact on the many species living in the regions. Polar bears, for example, need ice so that they can hunt for seals. A loss of sea ice could make it harder for these animals to acquire enough food. Pregnant females and those with cubs may be particularly at risk. The species of seal that need ice for resting or pup rearing will also be at risk.
As mentioned above, the change of the thermohaline circulation is expected to alter the speed and patterns of ocean currents, which in turn will impact fish stocks. According to the Third Assessment Report of the IPCC: “Arctic fisheries are among the most productive in the world. Changes in the velocity and direction of ocean currents affect the availability of nutrients and the disposition of larval and juvenile organisms, thereby influencing recruitment, growth and mortality.” Changes in the fish stocks are already taking place, with the result that some stocks are boosting while others are damaged. For example, Groundfish stocks have shown a positive response to recent climate change, while the thermally sensitive Greenland turbot and King crab stocks in the eastern Bering Sea and Kodiak are declining. The IPCC anticipates that harvests might halve or double depending on the stocks concerned; meanwhile some fisheries might die out altogether while new ones develop. This could increase or decrease local economies by hundreds of millions of dollars annually (IPCC). These projected changes in the abundance and distribution of fish stocks and wildlife are just one of the issues facing fishermen, Arctic communities and the indigenous people.
The Polar Regions annually accumulate pollution, including Persistent Organic Pollutants (POPs), which have been discharged from industry and agriculture around the globe. Melting of the sea ice could trigger the release of these pollutants which could cause them to re-enter the food chain. This would pose a health threat to top predators, e.g. polar bears and humans in these regions.
On the other hand, the melting of sea ice could have one economic benefit, namely in the area of shipping. The northwards retreat of sea ice opens up the Northern Sea Route, which would allow vessels to sail from Europe to the Far East by going north of Russia rather than using the existing route, i.e. the Suez Canal. Although this should reduce sailing times, cutting the costs of goods and air pollution, the risk of accidents leading to oil spills still remains.
Overview of impacts for coastal regions
The major impact for coastal regions caused by a change in the thermohaline circulation will be rising sea levels. There are many coastal sensitive regions around the globe which will be affected by even a small change in the sea level. As indicated above, the changing circulation can rise sea levels by either: melting of sea ice and ice sheets in the Polar Regions or via thermal expansion of the sea water. In 2007 approximately 634 million people lived in coastal areas within 9.1m of the sea level. Two thirds of the world’s cities with more than five million people are located in these low-lying coastal areas.
The IPCC Working Group II report indicates that current and future climate change would be expected to have a number of impacts, especially on coastal systems. Potential impacts may include: increased coastal erosion, higher storm surge flooding, inhibition of primary production processes, more extensive coastal inundation, changes in surface water quality and groundwater characteristics, increased loss of property and coastal habitats, increased flood risk and potential loss of life, loss of non-monetary cultural resources and values, impacts on agriculture and aquaculture via a decline in soil and water quality, and loss of tourism, recreation and transportation functions. Furthermore the IPCC report concludes that due to the great diversity of coastal environments; regional and local differences in projected relative sea level and climate changes; and differences in the resilience and adaptive capacity of ecosystems, sectors and countries, the impacts will be highly variable in time and space and will not necessarily be negative in all situations.
See also
Related articles
External Links
- Wikipedia: http://en.wikipedia.org/wiki/Thermohaline_circulation
- Ocean Motion and Surface Currents: http://oceanmotion.org/index.htm
- IPPC: http://www.ipcc.ch/
- UNEP: http://unep.org
References
- ↑ Broecker, W., 1991. The great ocean conveyor. Oceanography 1, 79–89.
- ↑ 2.0 2.1 2.2 2.3 2.4 2.5 2.6 2.7 2.8 Seidov, D., Barron, E., Haupt, B. J., 2000. Meltwater and the global ocean conveyor: northern versus southern connections. Global and Planetary Change 30, 257-270.
- ↑ , 1998. Asynchrony of Antarctic and Greenland climate change during the last glacial period. Nature 394, 739–743.
- ↑ Broecker, W.S., 1994. Massive iceberg discharges as triggers for global climate change. Nature 372, 421–424.
- ↑ Stocker, T.F., 1998. The seesaw effect. Science 282, 61–62.
- ↑ Vidal, L. et al., 1999. Link between the North and South Atlantic during the Heinrich events of the last glacial period. Clim. Dyn. 15, 909–919.
- ↑ 7.0 7.1 Birchfield, G.E., Broecker, W.S., 1990. A salt oscillator in the glacial Atlantic? 2. A scale analysis model. Paleoceanography 5, 835–843.
- ↑ Broecker, W.S., 2000. Was a change in thermohaline circulation responsible for the Little Ice Age? Proc. Natl. Acad. Sci. 97 (4), 1339–1342.
- ↑ Levitus, S., Antonov, J.I., Boyer, T.P., Stephens, C., 2000. Warming of the world ocean. Science 287, 2225–2229.
- ↑ 10.0 10.1 10.2 Geo Year Book 2004/2005: An overview of our changing environment. United Nations Environment Program. 80-84.
- ↑ Lemke, P., J. Ren, R.B. Alley, I. Allison, J. Carrasco, G. Flato, Y. Fujii, G. Kaser, P. Mote, R.H. Thomas and T. Zhang, 2007: Observations: Changes in Snow, Ice and Frozen Ground. In: Climate Change 2007: The Physical Science Basis. Contribution of Working Group I to the Fourth Assessment Report of the Intergovernmental Panel on Climate Change [Solomon, S., D. Qin, M. Manning, Z. Chen, M. Marquis, K.B. Averyt, M. Tignor and H.L. Miller (eds.)]. Cambridge University Press, Cambridge, United Kingdom and New York, NY, US, Pp. 375
- ↑ Godfrey, J.S., Love, G., 1992. Assessment of sealevel rise, specific to the South Asian and Australian situations. Seal Level Changes: Determination and Effects. Geophys. Monogr., vol. 69. AGU, Washington, DC, pp. 87–94.
- ↑ Church, J.A., Godfrey, J.S., Jacket, D.R., McDougall, T.R., 1991. A model of sealevel rise caused by ocean thermal expansion. J. Clim. 4, 438–456.
Please note that others may also have edited the contents of this article.
|