Open ocean habitat
This article describes the habitat of the Open oceans. It is one of the sub-categories within the section dealing with biodiversity of marine habitats and ecosystems. It gives an overview about the characteristics, zonation, biology and threats of the open oceans. Some legal aspects are also indicated.
Contents
Introduction
The open oceans or pelagic ecosystems are the areas away from the coastal boundaries and above the seabed (Fig. 1). It encompasses the entire water column and lies beyond the edge of the continental shelf. It extends from the tropics to the polar regions and from the sea surface to the abyssal depths. It is a highly heterogeneous and dynamic habitat. Physical processes control the biological activities and lead to substantial geographic variability in production.
The open ocean has a major impact on the climate of the earth. It has a stabilizing and balancing influence on the temperature, since currents are transporting energy in form of heat from the equatorial regions to the other parts of the globe. It is the main source of water in the atmosphere and a sink for carbon dioxide and hence, reduce the global greenhouse effect. Open seas are influencing regional and local weather events. Winds coming from open seas, usually bring rain. Typhoons come into existence as a result of differences in temperature between oceans and the atmosphere. Open seas serve as habitat to a highly specialized community of pelagic organisms and feeding ground for others.[1]
Zonation
The water column is subdivided into distinct zones by water depth and distance from shore. This is based on water depth and population composition. The distinct zones are (Fig. 2):
- The epipelagic zone ranges from the sea surface to a depth of about 200 metres. This is also the limit of the photic zone. Light penetrates into this surface water and is usually enough for the photosynthesis.
- The mesopelagic zone lies underneath the epipelagic zone and extends to about 1,000 metres.
- The bathypelagic zone is the zone between the 1,000 and 4,000 metres.
- The abyssalpelagic zone extends to a water depth of 6,000 metres.
- The hadalpelagic zone is deeper than 6,000 metres and is found in deep-sea trenches.
Characteristics
Currents
Currents are driven by differences in density, by wind action and by tides. Density is dependent on the temperature and the salinity of the water, see Seawater density. Cold and salty water is dense and will stay near the bottom, whereas warm and less salty water will stay near the surface. Cooling, warming, evaporation and freezing influence density differences between water masses and thus their relative motion. The circulation patterns driven by wind and density differences are strongly influenced by topography. Further information can be found in the article about large scale ocean circulation.
The largest ecosystems in the ocean are the subtropical gyres of the Atlantic, Pacific, and Indian Oceans. The North Pacific Subtropical Gyre (NPSG) ecosystem is the largest circulation feature on the planet and one of the most persistent, its boundaries having remained approximately the same for the past 10 million years. The NPSG has a clockwise circulation of less than 4 cm/s and forms a circumscribed, stable, and relatively homogenous habitat[2].
Light transmission and absorption

Life depends directly or indirectly on energy from sunlight. In the ocean, marine plants and protists (eukaryotic, single-celled organisms) use green chlorophyll and a few accessory pigments to capture the visible light from the sun. A large fraction of that sunlight is reflected from the sea surface back to the atmosphere. The remaining light enters the water and is absorbed by water molecules. Approximately 65% of the visible light in water is absorbed within 1 meter of the sea surface. This energy is converted into heat and elevates the surface water temperature. The red and yellow light (longer wavelengths) are absorbed by water more readily then the green and blue light (shorter wavelengths), see Fig. 3. This is called the selective absorption of wavelengths. It accounts for the blue colour of the open ocean. In very clear water, not even 1% of the light that enters the ocean penetrates to a depth of 100 meters. Due to the characteristics of light transmission, the water column can be divided into two distinct zones. The upper zone is called the photic zone. In this zone, plants receive sufficient sunlight and can photosynthesize. The zone below the photic zone is called the aphotic zone. Plants cannot survive in this dark zone. Water in the nearshore zone is generally turbid due to a high concentration of suspended solids. In this zone, light cannot penetrate deeper than 20 meters. The reflection by suspended particles causes a shift from blue to the green and yellow wavelengths.
Stratification
The water column is stratified by density differences related to temperature and salinity gradients, as explained in the article Seawater density. The interface zone between the less dense surface layer and the denser underlying water is called pycnocline. In the oceans, the pycnocline often coincides with the thermocline, that separates the warm, buoyant surface layer from the colder, denser deep layer (Fig. 4). The water above the thermocline is well mixed by the wind. Therefore the temperature in this water layer changes with the seasons, as well as the community of organisms. Besides the temperature, stratification can also be caused by differences is salinity. In this case, the transition zone for the salinity is called halocline.
In the ocean at low and mid latitudes (especially the subtropical gyres), the surface mixed layer ranges from 40 m to 100 m depth and is characterized by surface temperatures of 24 °C or higher. Large density differences suppress mixing of waters across the pycnocline. Stratification therefore separates the warm, sunlit surface waters from colder, nutrient-rich interior waters. This reduces the supply of nutrients, which become the limiting factor for primary productivity. This explains the very low surface chlorophyll concentrations (Fig. 5). High chlorophyll concentrations at the eastern margins of ocean basins (especially near western Africa) are caused by upwelling currents (see Ekman transport).
In the high-latitude ocean, the surface waters are cold and the vertical density gradient is therefore weak, allowing vertical mixing of waters to depths much greater than the sunlit euphotic zone. As a result, the nutrient supply is greater than the phytoplankton can consume, given the limited available light (and iron). Chlorophyll concentrations in the high-latitude ocean are much higher than in the subtropical ocean.
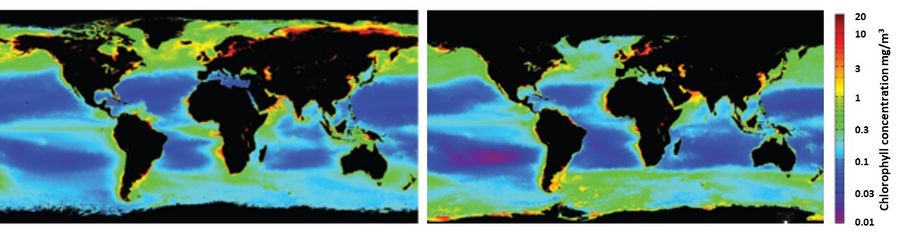
Biology
The epipelagic or photic zone
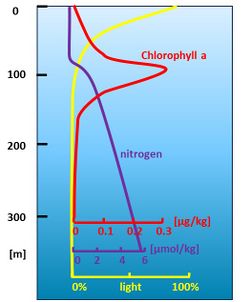
Throughout most of the coastal and open ocean, primary production is limited by the availability of inorganic nitrogen. Nitrogen fixation by cyanobacteria is an important source of nitrogen in the open ocean. Nitrogen fixating cyanobacteria need iron, which is carried as dust by winds from land to the ocean. Limitation of primary production by lack of iron is especially noticeable in the South Pacific[5].
Due to nitrogen deficiency, primary production is low in the sunlit upper ocean layer. However, near the bottom of the photic layer, nitrogen is supplied by turbulent diffusion from the mesopelagic layer below. This mesopelagic layer is much richer in nutrients due to the mineralization of sinking organic matter ('marine snow') released from the productive zone above. Most primary production therefore occurs in this productive zone, near the bottom of the photic layer, where light and nutrients are just sufficient (Fig. 6).
Despite the enormous size of the open ocean, it does not support a dense population of organisms in its water or on the seabed. Although the biomass is limited, species diversity is remarkably high. The pelagic flora falls into two main groups: the unicellular microphytoplankton and the large free-floating macroalgae. Microphytoplankton consists of diatoms, dinoflagellates and coccolithophores. The larger diatoms are mainly grazed by large zooplankton and these in turn are eaten by fish. Herbivorous zooplankton consists mainly of foraminifera and radiolarians. These are eaten by larger animals such as copepods. The floating organisms (neuston) at the surface have a blue colour due to the presence of protective pigments. These pigments are able to reflect the harmful part of the light spectrum (UV). This is necessary because the habitat is exposed to high levels of ultraviolet radiation. Organisms in the epipelagic zone such as crustaceans adopt red colours as a means of camouflage. This is possible because red light is quickly absorbed and does not penetrate far into this zone. The red colors are effectively invisible at depth and this makes the organisms invisible to others.[6] The epipelagic biome hosts a specialized fish fauna, including flying fishes and their relatives (needlefishes, halfbeaks), flotsam/jetsam-associated fishes (e.g., filefishes, triggerfishes, jacks, dolphinfish), 'baitfishes' (e.g., herrings, anchovies), larval/juvenile fishes of astounding variety (e.g., eels, flatfishes, snappers, to name a few) and large, highly migratory fishes (e.g., tunas, billfishes, mako sharks).[7]
![]() Fig. 7a. Neuston Physalia physalis[8] |
![]() Fig. 7c. Copepod [9] |
The mesopelagic or dysphotic zone
The dysphotic zone is a zone with very sparse light. The lower limit lies at a depth of approximately 500 to 1,000 meters. In this layer, oxygen levels can be low. These oxygen minima are caused by the bacterial breakdown of organic material sinking from the sea surface (see also Possible consequences of eutrophication). No seasonal effects of heating and cooling are present. This makes the zone stable and unchanging over time. The most abundant organisms are crustaceans (copepods, shrimps, amphipods, ostracods and prawns, Fig. 7). These organisms have red to red-orange pigmentation. Other animals are squids and fishes. Several adaptations have evolved to this zone. The first one is the presence of large size and light-sensing ability of the eyes. Some organisms have additional organs called photophores, which produce bioluminescence (Fig. 8). Some fish have bacterial photophores in which light is produced by the metabolic activities of symbiotic bacteria that live in dense concentrations in the photophores. Some species engage in diurnal vertical migration to get food. They swim upward to the photic zone at night to feed and descend again during the day. [6]
The bathypelagic or aphotic zone
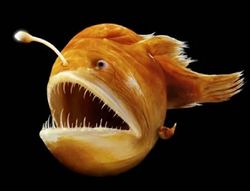
In the aphotic zone, it is always dark and cold. The zone begins at depths of 500 to 1,000 meters. Many species in this region are coloured red or black. Organisms that occur here are copepods, ostracods, jellyfishes, prawns, mysids, amphipods, worms and fishes. Fishes at mid-depth have adaptations evolved to maximize their chances of capturing their prey. These fishes are small with enormous mouths lined with sharp teeth. Their jaws can be unhinged to eat large preys. They also have bioluminescent organs. Some species have a fishing rod with an attached luminous lure in front of the head. This is the case for the female deep sea angler fish (Fig. 9). The body musculature is reduced. Many deep-sea species, including most fish species, are relatively slow growing, long lived, and late in maturation.
The deeper zones are dominated by macrobenthos. The principle food resources on the deep-sea bottom appear to be the slow fallout of fin and coarse organic detritus from surface waters, the settling of large animal carcasses, the sinking of fecal matter and the transport of organic detritus by turbidity currents. Bacteria in this region break down organic matter into simpler inorganic nutrients. The bacteria are consumed by heterotrophic nanoflagellates, which are in turn consumed by ciliates. This food chain is called microbial loop. This loop recycles organic matter that is too small to be consumed by metazoan plankton.
The greater the depth, the more organisms are confronted with increasing physiological stress. One of these stress factors is pressure. Pressure increases by 1 atmosphere for every 10 meters increase in depth. [10]
Threats
- Overfishing. Despite the fact that much of the open ocean is remote from the land, it has not escaped human impacts. To illustrate, 90% of the stocks of large pelagic fish, such as tuna and jacks, have been removed by fishing. The result of this overfishing is that the fishes of higher trophic levels are replaced by fishes of lower trophic levels. This is called fishing down the marine food web. It is ecologically unsustainable. More about this subject can be found in the articles on Effects of fisheries on marine biodiversity and Overexploitation.
- Pollution. This causes the loss of many species or a degradation of the environment, see the article Coastal pollution and impacts.
- Alien species. These are species that are introduced from another area and can compete with the indigenous species. This introduction can be done through ballast water from cargo ships or on hulls of vessels. More specific information is given in the article Non-native species invasions.
- Global warming. The direct effect of global warming is strongest for the animals at the top of the food web and much less for the lower trophic levels. However, the intermediate trophic levels (zooplankton) are strongly influenced by the indirect effect of cascading trophic interactions from the top of the food web. [11]
Legal aspect
In the United Nations Convention on the Law of the Sea (UNCLOS 1982), the definition of the open ocean or High Sea is: ‘The high seas are open to all States, whether coastal or land-locked. Freedom of the high seas is exercised under the conditions laid down by this Convention and the rules of international law. It comprises, inter alia, both for coastal and land-locked States:
- freedom of navigation
- freedom of overflight
- freedom to lay submarine cables and pipelines
- freedom to construct artificial islands and other installations permitted under international law
- freedom of fishing
- freedom of scientific research
No State may validly purport to subject any part of the high seas to its sovereignty. Every State shall effectively exercise its jurisdiction and control in administrative, technical and social matters over ships flying its flag. The States have prohibitions such as the transport of slaves, piracy, whaling and pollution. The States have to ensure the conservation and management of natural living resources. The high seas shall be reserved for peaceful purposes. Every State has the right to ships flying its flag on the high seas. Warships on the high seas have complete immunity from the jurisdiction of any State other than the flag State. Ships owned or operated by a State and used only on government non-commercial service shall have complete immunity from the jurisdiction of any State other than the flag State.’ [12]
For more details, see Legislation for the sea.
Related articles
References
- ↑ Göltenboth, F., Schoppe, S. and Widmann, P. 2006. Open ocean. In: Ecology of Insular SE Asia -The Indonesian Archipelago. Elsevier, pp. 74-81
- ↑ Grassle, J.F. 2001. Marine ecosystems. Encyclopedia of Biodiversity, Volume 4. Academic Press
- ↑ http://disc.sci.gsfc.nasa.gov/oceancolor/scifocus/oceanColor/oceanblue.shtml
- ↑ Sigman, D. M. and Hain, M. P. 2012. The Biological Productivity of the Ocean. Nature Education Knowledge 3(10), 21
- ↑ Falkowski, P. G., Barber, R. T. and Smetacek, V. 1998. Biogeochemical controls and feedbacks on ocean primary production. Science 281: 200–206
- ↑ 6.0 6.1 Pinet P.R. 1992. Oceanography: An introduction to the Planet Oceanus. West Publishing Company. p. 571
- ↑ Sutton, T.T. and Milligan, R.J. 2019. Deep-Sea Ecology. Encyclopedia of Ecology, 2nd edition, Vol. 1, pp. 35-45
- ↑ http://commons.wikimedia.org/wiki/Image:Portuguese_Man-O-War_(Physalia_physalis).jpg
- ↑ http://en.wikipedia.org/wiki/Copepod
- ↑ Kaiser M. et al. 2005. Marine ecology: Processes, systems and impacts. Oxford University Press. p.584
- ↑ Murphy, G.E.P., Romanuk, T.N. and Worm, B. 2020. Cascading effects of climate change on plankton community structure. Ecology and Evolution 10: 2170–2181
- ↑ http://www.un.org/Depts/los/convention_agreements/texts/unclos/closindx.htm
Please note that others may also have edited the contents of this article.
|