Plastics in the ocean
Since the 1950s, huge amounts of plastic have been produced worldwide. Plastic is a collective name for certain synthetic polymers, mainly polyurethane (PUR), polyethylene (PE), polyamide (PA), polyethylene terephthalate (PET), polystyrene (PS), polyvinyl chloride (PVC) and polypropylene (PP). Part of the plastic waste ends up in the oceans. This waste consists of plastic debris visible with the naked eye ('macroplastics' > 2.5 mm and 'mesoplastics' > 1 mm, according to the GESAMP expert group[1]) and microscopic pieces. At an international research workshop hosted by NOAA on the effects and fate of microplastic marine debris, participants suggested an upper size limit of 5 mm for microplastics[2]. Microplastics (MP) are particles that are either originally manufactured at micro size (primary MP) or are the result of physical, chemical and biological weathering and fragmentation of macroscopic debris (secondary MP). The smallest plastic fragments (< 1 μm) are called nanoplastics.
Microplastics occur in various shapes:[3]
- fragments - irregularly shaped pieces that result from the break-down of larger plastic items
- fibers - thin, elongated strands often originating from synthetic textiles or fishing nets
- beads - small, spherical particles commonly found in personal care products like exfoliating scrubs and toothpaste
- foams - lightweight, porous particles originating from products like polystyrene foam
- films - thin, flat pieces that result from the degradation of plastic bags, wrappers, and similar items
- pellets - pre-production plastic beads used in manufacturing, often spilled and dispersed into the environment
Many studies have been conducted to determine the amount of plastic in the oceans and the effects on the marine ecosystem. This article summarizes some important results of these studies.
Contents
[hide]Budget of plastics in the ocean
The current (2024) global production of plastics is estimated at about 400 million tons. About 8-9 % of fossil fuels consumed annually are used in the manufacturing of plastics (4–5% as feedstock and 3–4% as energy[4]). Polyethylene (PE), polypropylene (PP), and polyvinyl chloride (PVC) are the most dominant plastics globally (80 % by weight). Products made of plastic or containing plastics eventually become waste. Most waste products are destroyed (incinerated) or stored in landfills, some are recycled and some are released in the natural environment (‘mismanaged’). A small fraction ends up in the ocean, mostly via rivers[5], but also via sea-based sources (mainly discarded fishing gear) and the atmosphere. About half of the plastic debris larger than 0.5 mm in the Great Pacific Garbage Patch originates from fishing gear[6]. Estimates of the amount of plastic waste entering the sea vary widely, from 0.5 Mton/yr to more than 10 Mton/yr [7][8][9][5]. The lower values likely underestimate the supply rates of plastics to the ocean. They do not include atmospheric inputs [10] and plastic fragments smaller than <300 μm [11]. Sea-based sources account for 10-20% of the total input of plastics[9].
There is also much uncertainty about the amount of plastic accumulated in the oceans since the mass production began in the 1950s. Early estimates for the total amount of plastic in the surface ocean water range from 0.1–0.8 Mton for microplastics and around 0.8 Mton for plastic macrodebris[12][13][9]. This is a very small amount compared to the total input of plastics to the oceans over the past 60 years. This total input has been estimated at around 25 Mton (4-5% of the mismanaged plastic waste generated in the period 1961-2017[9]), but could be an order of magnitude larger[11][14].
There is compelling evidence from observations and model simulations that the majority of the plastic waste entering the sea does not (directly) reach the global ocean, but is retained in the coastal zone[15][13][16]. Large debris are broken down by mechanical fracturing in high-energy coastal and shelf environments during bedload transport and buried in sediments. Buoyant plastic debris generally become stranded on beaches[17]. Another part of the plastic waste (estimated at about 3-11 Mton[18]) consists of microdebris accumulated on the ocean floor through the incorporation of plastics into sinking organic particles (e.g., marine snow and fecal material)[14].
Micro- and nanoplastics
Recent studies attribute the major contribution to ocean plastic pollution to smaller size classes of microplastic and nanoplastic. Using nets with a mesh size of 100 μm resulted in 2.5-fold and tenfold higher microplastic concentrations than with 333 μm and 500 μm meshes, respectively[19]. Samples from the 200 m surface layer of the Atlantic ocean were analyzed for the three polymer groups polyethylene, polypropylene and polystyrene. The majority of the microplastics were <100 μm, with the peak size distribution observed in the range 50–75 μm for all the polymer groups. Only a small fraction of all three polymer groups were >300 μm. The three polymer groups represented a weight estimated at 11.6–21.1 Mton for the whole Atlantic ocean[11]. According to these figures, concentrations of micro and nanoplastics in the oceans fall in the range 0.01-10 mg/m3.
Micro- and nanoplastics are likely to originate mainly from land-based sources: effluents from wastewater treatment plants (e.g. components of cosmetic and care products, fibres from washed synthetic clothing), grindings from synthetic materials (e.g. tyre wear, carpet wear) transported by run-off or airborne, weathering of plastic waste along beaches that is washed to sea, etc. [20] Breakdown of microplastic debris in the ocean into nano-fibers is extremely slow and therefore considered less important[21]. Several studies report that the smaller the plastic particles, the more toxic they are to marine biota.[22]
Degradation of plastics in the marine environment
Degradation mechanisms for macroplastics occurring in the marine environment are: (i) colonization of plastics by microorganisms that form a biofilm on the polymer surface and induce biodegradation via surface erosion; (ii) depolymerization by abiotic hydrolysis of functional groups (esters, carbonates and amides), which causes molecular weight reduction; and (iii) photodegradation by exposure to UV light, resulting in molecular weight reduction and cracking of the material[23]. Microbes that degrade plastics attack plastics extracellularly to (partially) depolymerize the complex and large molecule into compounds that can be used as carbon and energy sources[24]. Fungi, like bacteria, can also use plastics as carbon source. Laccase enzymes play an important role in fungi attachment[23]. However, the energy yield of enzymatic degradation of microplastics is low, compared to the energy that can be retrieved from other carbon sources. The selective advantage for microbes to degrade microplastics is limited. This may explain why no significant adaptation of the ocean microbiome has occurred in spite of ubiquitous micro- and nanoplastics[25].
The worldwide most produced synthetic polymers with a pure carbon backbone (including polypropylene (PP), polyethylene (PE), polyvinyl chloride (PVC) and polystyrene (PS)) are unlikely to be degraded by microbes. No enzymes are known that act on these polymers[26][27]. Microbial degradation requires (artificially) aged polymer since physicochemical ageing processes increase the abundance of monomers and oligomers such that they may be degraded by microbial activity.
Weathering and photo-oxidation are generally considered to be the most important processes for the degradation of plastics. They result in a change in the chemical, physical and mechanical properties of the plastics and included chemical additives[28]. Abiotic factors, such as shear stress caused by wave action, ultraviolet radiation from the sun and heat make plastics brittle and break them up. Fragmentation of macroplastics results in micro- and nanoparticles. UV photodegradation breaks the polymer backbone of exposed plastics down, releasing low molecular-weight dissolved organic matter like carboxylic acids, monomers and more complex hydrocarbons or halogenated compounds[29]. These degradation products of UV radiation can be further decomposed by microbes. Chemical additives can also be a target for microbial degradation but may interfere with enzyme degradation processes[26]. Polystyrene can be completely mineralized by photooxidation[30].
Photodegradation is one of the major driving forces inducing the disintegration of plastics in the environment, including the reduction of chain length, surface oxidation, formation of microplastics and loss of mechanical properties[29]. It can be a key mechanism for fragmentation of plastic debris floating in the oceans. However, photo-oxidative fragmentation of plastic debris in the upper ocean layer is inhibited by several factors: short residence times before sinking, attenuation of UV radiation below the upper 1 meter, fouling by biota, low water temperature and low dissolved oxygen. This suggests that most of the abundant micro- and nanoplastics found in the oceans originate from primary sources (e.g. cosmetic products, washing/grinding of synthetic materials) or from photodegradation of macroplastic debris on land (especially stranded along shores) prior to reaching water[13][31].
Effective microbial degradation has been demonstrated for heterochain plastic polymers such as polylactic acid (PLA), polycaprolactone (PLC), polyurethane (PU) and polyethylene terephthalate (PET). These polymers contain oxygen, nitrogen or other heteroatoms in their backbone structure, which increases the susceptibility to degradation by biotic and abiotic hydrolysis reactions[27]. Bacteria found in the marine environment in association with polyethylene terephthalate (PET) and ester-based polyurethane (PUR) possess genes encoding monooxygenases, peroxidases and dehydrogenases, which can facilitate the initial degradation of these plastics[24]. However, currently known PUR- and PET-active enzymes and microorganisms have a very low conversion rate (on the order of decades to centuries[32]). Even for plastics marketed as biodegradable, such as PLA, prior treatment (such as heating) is necessary to accelerate microbial degradation[27].
Yoshida et al. (2016[33]) discovered that the bacterium Ideonella sakaiensis produces enzymes able to degrade polyethylene terephthalate (PET), using it as a carbon and energy source. However, only low-crystallinity PET can be degraded this way, whereas most PET water bottles have a high crystalline content which is not amenable to microbial degradation without prior treatment[34]. Another bacterium, Pseudalkalibacillus sp. MQ-1, has shown skill to increase the hydrophilicity of polyethylene and to decrease the crystallinity[35].
Ecosystem impacts
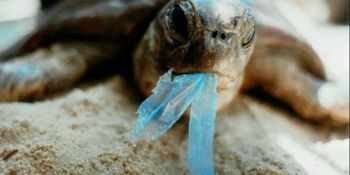
Some important observed impacts of plastics on marine ecosystems and fauna are:
- Macroplastics cause smothering of organisms when getting trapped in coastal ecosystems such as corals and mangroves. For example, corals can be detached from the hard substrate, with broken or dead branches. Exposure to light, oxygen exchange, prey capture and growth can be impaired and pathogens can get a foothold for invasion. On the other hand, plastic debris can provide a substrate for colonization by corals[36].
- Macroplastics can cause entanglement of marine animals. This happens especially for abandoned fishing gear (74% of the cases). Entanglements causing the amputation of limbs have been reported in all species of sea turtles. Entanglement with and ingestion of plastic debris have been reported in 35 species including dolphins, whales, seals, sea lions, sea otters, polar bears and manatees[36].
- The ingestion of macroplastics or microplastics can cause injury to the intestines of species and accumulate in the animal's organs, causing blockages of the gut tract that result in pseudo-satiety sensation and physiological stress, alteration of the feeding and retardation of growth, reduction in fertility, fecundity and survival rate of progeny[37]. A compilation of a large number of studies shows that on average 39% of all examined seabirds had ingested macroplastics, and on average 33% microplastics, while ingested microplastics where found in all sea turtles sampled in the Atlantic, Mediterranean and Pacific[36]. (Substantially lower percentages were reported in another literature survey[38]) There is evidence of the ingestion of microplastics by marine zooplankton, as well as the transfer of microplastic particles from mesozooplankton to macrozooplankton. Therefore a real risk exists of microplastics invading marine food webs[39]. Microplastics impair the capability of fish larvae to evade predators[40]. As these organisms are more likely to be eaten, the concentration of microplastics in their predators will be biomagnified[41]. As an example of biomagnification, a krill-feeding blue whale consumes 2.51 to 43.6 kg of microplastics per day[42].
- Micro and nanoplastics can cross cell membranes[26]. Adverse effects have been observed in many organisms exposed to synthetic fibres, particularly in species at the lower end of the food chain. Laboratory studies on the exposure of marine biota to microplastics report, among other things, reduced fitness of zooplankton populations, reduced filtration rates of bivalves, increased mortality in crabs and gill damage in fish, together with increased oxidative stress and altered metabolism[43].
- Chemical additives or pollutants adsorbed to plastic debris from seawater can become bioavailable when ingested by organisms. Adsorbed contaminants such as pesticides, herbicides and heavy metals (e.g. Al, Cd, Co, Cr, Cu, Fe, Hg, Mn, Pb and Zn) can lead to changes in metabolic and reproductive activity, impaired immune response, oxidative stress, cellular or subcellular toxicity, inflammation and cancer[37]. Micro- and nanoplastics have a high surface area to volume ratio and can therefore adsorb greater amounts of hydrophobic contaminants from the environment than macroplastics. They can also be taken up by a much wider range of species, so their potential to enter marine food webs and move up trophic levels through predation is higher. Especially filter-feeding animals, from small mussels to large whale sharks, can ingest considerable amounts of micro- nanoplastics[36]. On the other hand, chemicals accumulated in the body can adsorb to microplastics and be excreted, reducing the burden on the organism. Similarly, plastics in water can accumulate chemicals, leading to a lower concentration of bio-available chemicals in the water[36].
Toxic leachates
During manufacture, plastics are commonly provided with additives to impart desirable and beneficial properties. In some cases, additives represent up to 30% of the plastic weight[27]. Release of these additives is boosted by UV weathering[29]. Frequently added chemicals include bisphenol-A (BPA), per- and polyfluoroalkyl (PFAS), brominated flame retardants, PCBs and a group of substances called phthalates[44]. For example, PFASs are added for water, heat, and grease resistance, while bisphenols are added for flexibility properties. These chemicals belong to the so-called endocrine disruptors, which disrupt the hormonal functions of animals (including humans). In zooplankton, exposure to PFAS yields developmental retardation, reduced fecundity, oxidative stress, irregular apoptosis, cellular proliferation, and generates reactive oxidative species (ROS) which further damages DNA[43]. Bisphenols have significant negative impacts on reproductive function in marine animals, where males experience more prominent and lasting effects. Other added endocrine disruptors have similar detrimental effects when they enter the gastrointestinal tract of organisms after leaching from ingested microplastics. They affect movement, behavior, sexual development, growth (including growth abnormalities), survival and reproduction. They can bioaccumulate in organisms such as fish and tadpoles. Early life stages, amphibians, fish and invertebrates are particularly sensitive[36]. Additives also include toxicologically relevant trace metals and metalloids such as As, Cd, Sb, Sn or Pb [29]
It must be noted that not all fibres in the environment are of synthetic origin. Fibres of natural origin (e.g. cotton, viscose) are also widespread. These natural fibres are often chemically treated with dyes, additives, and flame retardants and therefore pose a similar threat[43].
To date (2022), the problem of micro- and nanoplastic effects in the marine environment has been assessed almost exclusively on the basis of laboratory exposure studies. These studies typically look at concentrations of micro- and nanoplastics that are much higher (up to g/l) than concentrations in the natural marine environment (< 10 µg/l) [45][3]. It was shown, for example, that at such high concentrations, plastic leachates impair photosynthesis in common picophytoplankton species, such as Prochlorococcus [46], whereas no effect of microplastics on photosynthesis was found in studies with naturally occurring concentrations[47]. Linking laboratory results to natural exposure levels is essential to avoid overestimation of the problem. Improvement of existing techniques is needed to obtain reliable and reproducible information on exposure levels in natural environments[48]. An overview of major current research results and gaps regarding the toxicity of microplastics is given by Ahmed et al. (2023[45].
Management options to reduce the plastic plague
Plastics are a commodity with many benefits and almost no equivalent alternatives. A huge amount of plastics is produced annually to meet the demand of the global population. A great drawback is the current pollution of the planet with plastic waste, caused by the (mostly unintended) release of plastics into the environment. Human behavior is at the root of the plastic plague.
The lifespan of plastics abandoned in the environment is very long. Plastics are hardly degraded by natural processes. Management options for dealing with the plastic plague must therefore address in the first place the causes of plastic release into the environment.
The major cause of plastic pollution is the careless discard of plastic items. The general public does not sufficiently realize that abandoned plastic is harmful waste. Raising public awareness through educating people about the negative impacts of plastic pollution should be a management priority. Education can be reinforced by legal regulations. As packaging is a major source of plastic waste (around 40% globally), putting a price on plastic package with the introduction of a deposit-refund system provides users with an incentive to collect plastic waste instead of leaving it in the environment. Facilitating the collection of plastic waste enhances the effectiveness of measures to change consumer behaviors. Appropriate techniques and regulations for processing and disposal of collected plastic waste are essential to prevent leakage to the environment.
Release of plastic waste into the environment can further be limited by imposing regulations on the production of plastics. Many countries have introduced a ban om single-use plastics.[49]
Given current technologies and practices, options for the application of recycled plastics are limited. Recycled plastics are currently expensive and of low quality, as most of the plastic waste consists of a mixture of different types of plastics[50]. Existing bioplastics do not solve the problem due to their limited degradability. Recycling of plastics can be greatly facilitated by standardized production practices, for example: [51]
- Restriction of the use of additives such as pigments and plasticizers
- Implementation of a traceability system that provides information about the composition of plastic, with visible labelling and standardized codes for automatic sorting at recycling facilities
- No combinations of polymers that are difficult to separate, such as polypropylene (PP) and polyethylene (PE) and no mixtures including PVC
- Preferential use of common plastics (such as PE and PP) in products to increase the volume of homogeneous material for recycling
- Use of recycled plastic in products to drive the demand and improve the market conditions for recycled plastic
The legally binding Extended Producer Responsibility (EPR) imposes obligations to manufacturers to design resource-efficient and low-impact products and to ensure effective end-of-life collection, the environmentally sound treatment of collected products and improved rates of reuse and recycling. The European Waste Framework Directive includes general minimum requirements to improve harmonization, increase transparency, cost-efficiency, accountability and better enforcement of EPR obligations at national level. Regulations for Producer Responsibility Obligations are also being implemented at national level in the UK while new EPR regulations in the USA are being implemented at state level.[49]
Research on plastic degradation (e.g. degradable plastics[52], genetically modified plastic-degrading microbes[53]) can contribute to mitigating the plastic waste plague, but have not yet yielded major breakthroughs.
Rivers are the main pathway of plastic pollution of the ocean. Because of the considerable retention time of plastics in rivers and estuaries, the release of plastics to the ocean can be reduced by cleaning up temporarily trapped plastic waste. However, reliable assessments of the effectiveness of current clean-up technologies are generally lacking[54]
Related articles
References
- Jump up ↑ Joint Group of Experts on the Scientific Aspects of Marine Environmental Protection (GESAMP) 2015. Sources Fate and Effects of Microplastics in the Marine Environment: A Global Assessment
- Jump up ↑ Arthur, C. D., Opfer, S. E. and Bamford, H. A. 2009. Standardization of field methods for collection of microplastic marine debris: pelagic, shoreline and sediment protocols. New Orleans, USA, 19-23 November 2009
- ↑ Jump up to: 3.0 3.1 Kushwaha, M., Shankar, S., Goel, D., Singh, S., Rahul, J., Rachna, K. and Singh, J. 2024. Microplastics pollution in the marine environment: A review of sources, impacts and mitigation. Marine Pollution Bulletin 209 (2024) 117109
- Jump up ↑ Nielsen, T.D., Hasselbalch, J., Holmberg, K., Stripple, J., 2020. Politics and the plastic crisis: a review throughout the plastic life cycle. Wiley Interdiscip Rev Energy Environ. 9, e360
- ↑ Jump up to: 5.0 5.1 Strokal, M., Vriend, P., Bak, M.P., Kroeze, C., van Wijnen, J. and van Emmerik, T. 2023. River export of macro- and microplastics to seas by sources worldwide. Nature Communications 14, 4842
- Jump up ↑ Lebreton, L., Slat, B., Ferrari, F., Sainte-Rose, B., Aitken, J., Marthouse, R., Hajbane, S., Cunsolo, S., Schwarz, A., Levivier, A., Noble, K., Debeljak, P., Maral, H., Schoeneich-Argent, R., Brambini, R. and Reisser, J. 2018. Evidence that the Great Pacific Garbage Patch is rapidly accumulating plastic. Sci. Rep. 8, 4666
- Jump up ↑ Jambeck, J.R., Geyer, R., Wilcox, C., Siegler, T.R., Perryman, M., Andrady, A., Narayan, R. and Law, K.L. 2015. Plastic waste inputs from land into the ocean. Science 347: 768–771
- Jump up ↑ Schmidt, C., Krauth, T. and Wagner, S. 2017. Export of plastic debris by rivers into the sea. Environ. Sci. Technol. 51: 12246–12253
- ↑ Jump up to: 9.0 9.1 9.2 9.3 Isobe, A. and Iwasaki, S. 2022. The fate of missing ocean plastics: Are they just a marine environmental problem? Science of the Total Environment 825, 153935
- Jump up ↑ Allen, D., Allen, S., Abbasi, S., Baker, A., Bergmann, M., Brahney, J., Butler, T., Duce, R. A., Eckhardt, S., Evangeliou, N., Jickells, T., Kanakidou, M., Kershaw, P., Laj, P., Levermore, J., Li, D., Liss, P., Liu, K., Mahowald, N., Masque, P., Materic, D., Mayes, A.G., McGinnity, P., Osvath, I., Prather, K.A., Prospero, J.M., Revell, L.E., Sander, S.G., Shim, W.J., Slade, J., Stein, A., Tarasova, O. and Wright, S. 2022. Microplastics and Nanoplastics in the Marine-Atmosphere Environment. Nature Reviews Earth & Environment 3: 393–405
- ↑ Jump up to: 11.0 11.1 11.2 Pabortsava, K. and Lampitt, R.S. 2020. High concentrations of plastic hidden beneath the surface of the Atlantic Ocean. Nat. Commun. 11, 4073
- Jump up ↑ van Sebille, E., Wilcox, C., Lebreton, L., Maximenko, N., Hardesty, B.D., van Franeker, J.A. and Eriksen, M., Siegel, D., Galgani, F. and Law, K.L. 2015. A global inventory of small floating plastic debris. Environ Res Lett 10, 124006
- ↑ Jump up to: 13.0 13.1 13.2 Lebreton, L., Egger, M. and Slat, B. 2017. A global mass budget for positively buoyant macroplastic debris in the ocean. Scientific Reports 9, 12922
- ↑ Jump up to: 14.0 14.1 Zhu, X., Rochman, C.M., Hardesty, B.D. and Wilcox, C. 2024. Plastics in the deep sea – A global estimate of the ocean floor reservoir. Deep Sea Research Part I: Oceanographic Research Papers 206, 104266
- Jump up ↑ Chenillat, F., Huck, T., Maes, C., Grima, N. and Blanke, B. 2021. Fate of floating plastic debris released along the coasts in a global ocean model. Marine Pollution Bulletin 165, 112116
- Jump up ↑ Harris, P.T. 2020. The fate of microplastic in marine sedimentary environments: A review and Synthesis. Marine Pollution Bulletin 158, 111398
- Jump up ↑ Onink, V., Jongedijk, C.E., Hoffman, M.J., Van Sebille, E. and Laufkotter, C. 2021. Global simulations of marine plastic transport show plastic trapping in coastal zones. Environ. Res. Lett. 16, 064053
- Jump up ↑ Harris, P.T., Maes, T. and Raubenheimer, K. 2023. A marine plastic cloud - Global mass balance assessment of oceanic plastic pollution. Continental Shelf Research 255, 104947
- Jump up ↑ Lindeque, P.K., Cole, M., Coppock, R.L., Lewis, C.N., Miller, R.Z., Watts, A.J.R., Wilson-McNeal, A., Wright, S.L. and Galloway, T.S. 2020. Are we underestimating microplastic abundance in the marine environment? A comparison of microplastic capture with nets of different mesh-size. Environ. Pollut. 265, 114721
- Jump up ↑ Mishra, S., Rath, C.C. and Das, A.P. 2019. Marine microfiber pollution: A review on present status and future challenges. Marine Pollution Bulletin 140: 188–197
- Jump up ↑ Andrady, A.L. 2022. Weathering and fragmentation of plastic debris in the ocean environment. Marine Pollution Bulletin 180, 113761
- Jump up ↑ Leistenschneider, C., Wu, F., Primpke, S., Gerdts, G. and Burkhardt-Holm, P. 2024. Unveiling high concentrations of small microplastics (11–500 μm) in surface water samples from the southern Weddell Sea off Antarctica. Science of the Total Environment 927, 172124
- ↑ Jump up to: 23.0 23.1 Viel, T., Manfra, L., Zupo, V., Libralato, G., Cocca, M. and Costantini, M. 2023. Biodegradation of Plastics Induced by Marine Organisms: Future Perspectives for Bioremediation Approaches. Polymers 2023, 2673
- ↑ Jump up to: 24.0 24.1 Vaksmaa, A., Hernando-Morales, V., Zeghal, E. and Niemann, H. 2021. Microbial Degradation of Marine Plastics: Current State and Future Prospects 5. Biotechnology for Sustainable Environment, Eds. S. J. Joshi et al. Springer Nature Singapore
- Jump up ↑ Gambarini, V., Drost, C.J., Kingsbury, J.M., Weaver, L., Pantos, O., Handley, K.M. and Lear, G. 2024. Uncoupled: investigating the lack of correlation between the transcription of putative plastic-degrading genes in the global ocean microbiome and marine plastic pollution. Environmental Microbiome 19, 34
- ↑ Jump up to: 26.0 26.1 26.2 Danso, D., Chow, J. and Streit, W.R. 2019. Plastics: Environmental and Biotechnological Perspectives on Microbial Degradation. Applied and Environmental Microbiology 85: 1095-1119
- ↑ Jump up to: 27.0 27.1 27.2 27.3 Lear, G., Maday, S.D,M., Gambarini, V., Northcott, G., Abbel, R., Kingsbury, J.M., Weaver, L., Wallbank, J.A. and Pantos, O. 2022. Microbial abilities to degrade global environmental plastic polymer waste are overstated. Environ. Res. Lett. 17, 043002
- Jump up ↑ Bridson, J.H., Masterton, H., Theobald, B., Risani, R., Doake, F., Wallbank, J.A., Maday, S.D.M., Lear, G., Abbel, R., Smith, D.A., Kingsbury, J.M., Pantos, O., Northcott, G.L. and Gaw, S. 2024. Leaching and transformation of chemical additives from weathered plastic deployed in the marine environment. Marine Pollution Bulletin 198, 115810
- ↑ Jump up to: 29.0 29.1 29.2 29.3 Menger, F., Romerscheid, M., Lips, S., Klein, O., Nabi, D., Gandrass, J., Joerss, H., Wendt-Potthoff, K., Bedulina, D., Zimmermann, T., Schmitt-Jansen, M., Huber, C., Bohme, A., Ulrich, N., Beck, A.J., Profrock, D., Achterberg, E.P., Jahnke, A. and Hildebrandt, L. 2024. Screening the release of chemicals and microplastic particles from diverse plastic consumer products into water under accelerated UV weathering conditions. Journal of Hazardous Materials 477, 135256
- Jump up ↑ Ward, C.P., Armstrong, C.J., Walsh, A.N., Jackson, J.H. and Reddy, C.M. 2019. Sunlight converts polystyrene to carbon dioxide and dissolved organic carbon. Environ. Sci. Technol. Lett. 6: 669–674
- Jump up ↑ Andrady, A.L. 2022. Weathering and fragmentation of plastic debris in the ocean environment. Marine Pollution Bulletin 180, 113761
- Jump up ↑ Krueger, M.C., Harms, H. and Schlosser, D. 2015. Prospects for microbiological solutions to environmental pollution with plastics. Appl. Microbiol. Biotechnol. 99: 8857–8874
- Jump up ↑ Yoshida, S., Hiraga, K., Takehana, T., Taniguchi, I., Yamaji, H., Maeda, Y., Toyohara, K., Miyamoto, K., Kimura, Y. and Oda, K. 2016. A bacterium that degrades and assimilates poly(ethylene terephthalate). Science 351: 1196–1199
- Jump up ↑ Wallace, N.E., Adams, M.C., Chafin, A.C., Jones, D.D., Tsui, C.L. and Gruber, T.D. 2020. The highly crystalline PET found in plastic water bottles does not support the growth of the PETase-producing bacterium Ideonella sakaiensis. Environ. Microbiol. Rep. 12: 578–582
- Jump up ↑ Meng , Q., Yi, X., Zhou, H., Song, H., Liu, Y., Zhan, J. and Pan, H. 2024. Isolation of marine polyethylene (PE)-degrading bacteria and its potential degradation mechanisms. Marine Pollution Bulletin 207, 116875
- ↑ Jump up to: 36.0 36.1 36.2 36.3 36.4 36.5 Tekman, M. B., Walther, B. A., Peter, C., Gutow, L. and Bergmann, M. 2022. Impacts of plastic pollution in the oceans on marine species, biodiversity and ecosystems. WWF Germany, Berlin, pp. 221
- ↑ Jump up to: 37.0 37.1 Guzzetti, E., Sureda, A., Tejada, S. and Faggio, C. 2018. Microplastic in marine organism: Environmental and toxicological effects. Environmental Toxicology and Pharmacology 64: 164–171
- Jump up ↑ Kibria, G. 2024. Contamination of coastal and marine bird species with plastics: Global analysis and synthesis. Marine Pollution Bulletin 206, 116687
- Jump up ↑ Ugwu, K., Herrera, A. and Gomez, M. 2021. Microplastics in marine biota: A review. Marine Pollution Bulletin 169, 112540
- Jump up ↑ Pannetier, P., Morin, B., Le Bihanic, F., Dubreil, L., Clérandeau, C., Chouvellon, F., van Arkel, K., Danion, M. and Cachot, J. 2020. Environmental samples of microplastics induce significant toxic effects in fish larvae. Environment International 134, 105047
- Jump up ↑ Hajji, A.L. and Lucas, K.N. 2024. Anthropogenic stressors and the marine environment: From sources and impacts to solutions and mitigation. Marine Pollution Bulletin 205, 116557
- Jump up ↑ Kahane-Rapport, S.R., Czapanskiy, M.F., Fahlbusch, J.A., Friedlaender, A.S., Calambokidis, J., Hazen, E.L., Goldbogen, J.A. and Savoca, M.S. 2022. Field measurements reveal exposure risk to microplastic ingestion by filter-feeding megafauna. Nat. Commun. 13, 6327
- ↑ Jump up to: 43.0 43.1 43.2 Concato, M., Panti, C., Baini, M., Galli, M., Giani, D. and Fossi, M.C. 2023. Detection of anthropogenic fibres in marine organisms: Knowledge gaps and methodological issues. Marine Pollution Bulletin 191, 114949
- Jump up ↑ Gallo, F., Fossi, C., Weber, R., Santillo, D., Sousa, J., Ingram, I., Nadal, A. and Romano, D. 2018. Marine litter plastics and microplastics and their toxic chemicals components: the need for urgent preventive measures. Environ Sci. Eur. 30, 13
- ↑ Jump up to: 45.0 45.1 Ahmed A.S.S., Billah, M.M., Ali, M.M., Bhuiyan, M.K.A., Guo,L., Mohinuzzaman,M., Hossain, M.B., Rahman, M.S., Islam, M.S., Yan, M.and Cai, W. 2023. Microplastics in aquatic environments: A comprehensive review of toxicity, removal, and remediation strategies. Science of the Total Environment 876, 162414
- Jump up ↑ Tetu, S.G., Sarker, I., Schrameyer, V., Pickford, R., Elbourne, L.D.H., Moore, L.R. and Paulsen, I.T. 2019. Plastic leachates impair growth and oxygen production in Prochlorococcus, the ocean’s most abundant photosynthetic bacteria. Commun Biol. 2019;2, 184
- Jump up ↑ Sjollema, S.B., Redondo-Hasselerharm, P., Leslie, H.A., Kraak, M.H.S. and Vethaak, A.D. 2016. Do plastic particles affect microalgal photosynthesis and growth? Aquat. Toxicol. 170: 259–261
- Jump up ↑ Piccardo, M., Renzi, M. and Terlizzi, A. 2020. Nanoplastics in the oceans: Theory, experimental evidence and real world. Marine Pollution Bulletin 157, 111317
- ↑ Jump up to: 49.0 49.1 Tumu, K., Vorst, K.and Curtzwiler, G. 2023. Global plastic waste recycling and extended producer responsibility laws. Journal of Environmental Management 348, 119242
- Jump up ↑ Verrips, A., Hoogendoorn, S., Jansema-Hoekstra, K. and Romijn, G. 2019. The Circular Economy of Plastics in the Netherlands. In: Environmental Sustainability and Education for Waste Management. Springer Nature Singapore
- Jump up ↑ Strömberg, E., Olofsson, K., Brännström, S. and Almasi, A. 2024. Design for recycling of products containing plastics. Pre-study: towards a global standard which contributes to plastics circularity. Swedish Environmental Research Institute, Report C825
- Jump up ↑ Afshar, S.V., Boldrin, A., Astrup, T.F., Daugaard, A.E. and Hartmann, N.B. 2024. Degradation of biodegradable plastics in waste management systems and the open environment: A critical review. Journal of Cleaner Production Volume 434, 140000
- Jump up ↑ Martín-González, D., de la Fuente Tagarro, C., De Lucas, A., Bordel, S. and Santos-Beneit, F. 2024. Genetic Modifications in Bacteria for the Degradation of Synthetic Polymers: A Review. Int. J. Mol. Sci. 25, 5536
- Jump up ↑ Griffin, M.D., Diana, Z.T., Karasik, R. and Dunphy-Daly, M.M. 2024. Do plastic clean-up technologies work? What research does (and doesn't) tell us. Marine Pollution Bulletin 209, 116978
Please note that others may also have edited the contents of this article.
|