Difference between revisions of "Effects of fisheries on European marine biodiversity"
Dronkers J (talk | contribs) |
Dronkers J (talk | contribs) |
||
Line 1: | Line 1: | ||
− | |||
Fishing is the most widespread human exploitative activity in the marine environment. Pauly and Christenen (1995) estimated that over 20 % of the [[primary production]] is required to sustain fisheries in many intensively fished coastal ecosystems.<ref name="Pauly1995">Pauly, D. & Christensen, V.(1995). Primary production required to sustain global fisheries. ''Nature'' 374: 255-257.</ref> | Fishing is the most widespread human exploitative activity in the marine environment. Pauly and Christenen (1995) estimated that over 20 % of the [[primary production]] is required to sustain fisheries in many intensively fished coastal ecosystems.<ref name="Pauly1995">Pauly, D. & Christensen, V.(1995). Primary production required to sustain global fisheries. ''Nature'' 374: 255-257.</ref> |
Revision as of 16:23, 7 November 2019
Fishing is the most widespread human exploitative activity in the marine environment. Pauly and Christenen (1995) estimated that over 20 % of the primary production is required to sustain fisheries in many intensively fished coastal ecosystems.[1]
Fishing has a number of direct effects on marine ecosystems because it is responsible for increasing mortality of target and by-catch species; an important physical impact on the habitat of benthic organisms is caused by bottom trawling. The direct effects of fishing have indirect implications for other species as well. Fisheries remove prey that piscivorous fishes, birds and mammals would otherwise consume, or may remove predators that would otherwise control prey populations. Reductions in the density of some species may affect competitive interactions and result in the proliferation of non-target species. The activities of fisheries also favour scavengers, they obtain more food by the discarded by-catch and because a range of species are killed, but not retained by towed gears.[2]
Direct effects of fishing
Direct effects on target species
Global landing of fish and other marine catches began stagnating in the early 1980s.[3] Biomass in the North Atlantic fell by 90 % during the twentieth century, leading to declines of catches throughout the North Atlantic, notably in eastern Canada. It has taken less than a century for North Atlantic fisheries to reduce the biomass of the high-trophic-level fishes to under 10% of their original amounts.[4]
Historically, fishing started at the top of most food chains by removing the highly valuable and more easily cacheable species, then moved down to the next biggest species as those above were depleted and were no longer easily or economically caught. The downward shift towards fish catches of lower trophic levels results in ‘fishing down the food web’. The mean trophic level of reported catches had declined over the years. For all marine areas, the trend has been a decline in the mean trophic level of the fisheries landings form slightly more than 3.3 in the early 1950s to less than 3.1 in 1994.[5]
Another shift in the global landings of fishes in the last 50 year is from shallow to deeper water species; this resulted in the fact that the mean longevity of the fish species caught, has increased dramatically. This trend is a serious concern because species with larger body size, longer life span, later sexual maturity and slow growth (e.g. Dogfish Scyliorhinus canicula, Rays, Conger eel Conger conger) are more vulnerable to overfishing.[6]
Direct effects on by-catch species
Benthic organisms and other unwanted by-catch are often discarded and a range of species are killed, but not retained by towed gears.
Some by-catch species have been affected dramatically by fishing. For example, the sizes of three dolphin populations (a Stenella longirostris population, a S. attenuata population and a second S. longirostris population) caught by tuna boats in the eastern tropical Pacific were reduced to 20%, 35-50% and 58-72% of pre-exploitation levels by 1997.[2]
Direct effects of physical disturbance
The direct effects of fishing, related to physical disturbance include the scraping, scouring and resuspension of the substratum. The effects vary according to the gears used and the habitats fished.[2]
It seems reasonable to predict that the effects of physical disturbance will be short-lived in communities adapted to frequent natural perturbations (e.g. a cockle community) in contrast to those communities found in habitats exposed to fewer disturbances (e.g. the abyssal plane).[2]
The changes associated with physical disturbance are relatively short-lived for the majority of small species, longer-lived organisms decolonize more slowly. For example, Beukema (1995) reported that the biomass of gaper clams, Mya arenaria L., took 2 years to recover after lugworm dredging in the Wadden Sea, whereas small polychaetes and bivalves had recolonized the dredged areas within 12 months.[2]
Communities dominated by long-lived suspension feeders are most likely to be replaced by a community of opportunistic deposit-feeding species and mobile epifauna when subjected to large-scale and intense fishing disturbance. More dramatically, biogenic structures that increase the complexity of the epibenthic habitat (e.g. worm tubes) create specialized environmental conditions by altering local hydrographic conditions that encourage the development of a specialized associated community. Loss of such structures will also affect the survivorship of any associated species and prolong the recolonisation process.[2]
A particular sensitive hard-bottom habitat is the deep-water coral (the basis for the reef formation is Lophelia pertusa) communities. These communities are mainly found at the offshore shelf edges of the Arctic and North-Atlantic ocean. Some offshore reefs have experienced considerable damage due to trawling activities. The Lophelia reefs have recently (since 1999) been protected from fishing activities by the Norwegian authorities.[7]
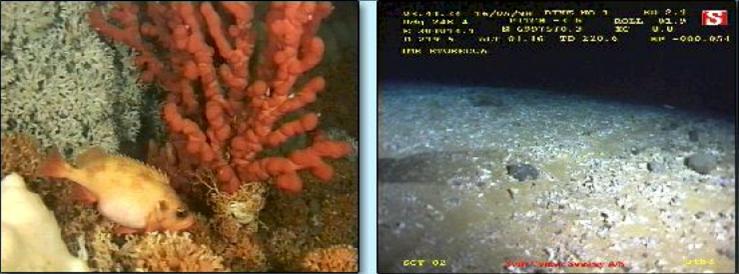
In soft mud communities a large proportion of the fauna live in burrows up to 2 m deep.[8] Few of these deep burrowing fauna are likely to be affected by passing trawls. However, the energetic costs of repeated burrow reconstruction may have long-term implications for the survivorship of individuals.
Sessile epibenthic species are most likely to be vulnerable to the passage of bottom gears. The disappearance of reefs of the calcareous tube building worm, Sabellaria spinulosa Leukart and their replacement by small polychaete communities, indicated that dredging activity had caused measurable changes in the Wadden Sea benthic community.[9]
Changes attributed to the fisheries are identified in the mesozooplankton composition. For instance, the mesozooplankton taken in continuous plankton recorder samples in the central North Sea were numerically dominated by calanoid copepods from 1958 to the late 1970s, whereas samples taken from the same stations from the early 1980s to early 1990s were dominated by the pluteus larvae of echinoid and ophiuroid echinoderms. This trend is consistent with the reported increases in the abundance of echinoderms in benthic communities which may have been stimulated, in part, by bottom trawling.[10]
Static bottom gears are anchored to the seabed and left to fish passively. The most commonly used are gill, trammel or tangle nets, which are designed to capture target species by enmeshing or tangling them.[11][12]
Net and pot fisheries are static, for this reason , the areas of seabed affected by each gear is insignificant compared with the widespread effects of mobile fishing gears. However, effort may be significant if concentrated in relatively small areas with communities of long-lived fauna (e.g. the foliose bryozoan Pentapora foliacea).
Indirect effects of fisheries
Effects of ‘ghost-fishing’
When nets or catch-pots are lost, they may continue to fish. This phenomenon is known as ‘ghost-fishing’. In circumstances where nets or pots are snagged onto rocks, holding the net in place, or lost in relatively stable deep water environments, they may continue to fish indefinitely. In these cases, a typical pattern of capture is observed. Over the first few days, catches decline almost exponentially as the increasing weight of catch causes the net to collapse. Then, for the next few weeks, the decaying bodies of fishes and Crustacea attract large number of scavenging crustaceans, many of which are valuable commercial species and also become entangled in the net. Thereafter, there appears to be a continuous cycle of capture, decay and attraction for as long as the net has some entanglement properties.[13]
Trophic cascading effect
Changes in one level of a food web can also have cascading effects on others. For example in the Black Sea, a trophic cascade has acted by fishery removals of apex predators (bonito Sarda sarda, mackerel Scomber scombrus , bluefish Pomatomus saltatrix, dolphins) which caused a decreased consumer control and lead to higher abundance of planktivorous fish (i.e. Black Sea sprat Clupeonella cultriventris, anchovy Engraulis encrasicholus, horse mackerel Trachurus mediterraneus ponticus). The increased consumption by planktivorous fish causes a decline in zooplankton biomass that in turns allowed phytoplankton to increase. This chain of events is thought to explain the explosions of phytoplankton and jellyfish reported in the Black Sea over the past 30 years.[14]
‘Food-web’ competition
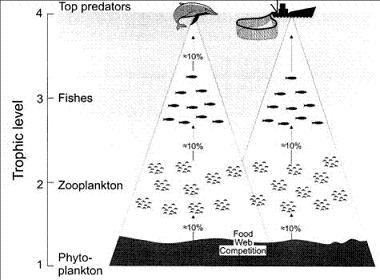
An apex predator may be affected by fisheries even when the prey and species caught do not overlap. This has been termed ‘food-web competition’.[15] Food-web competition occurs when there is potential overlap of the trophic flows supporting a given group (e.g. marine mammals) with the trophic flows supporting another group (e.g. fisheries). The relationship between the size of fishery catches and the amounts of primary production required to sustain fisheries and marine mammals suggests that the primary production available to marine mammals may decline as catches increase.[4]
Effects on phenotypic evolution
Large changes in size-at-age and age-at-maturation of commercially exploited fish have been reported in a number of ecosystems. Survival and reproduction are functions of body size. Small fish generally incur higher mortality rates and produce fewer eggs than larger fish.
Gear is designed to remove some kinds of individuals in preference to others, usually individuals that are larger and, indirectly, older. The location of fishing is often non-random relative to spatial distributions of stocks, being concentrated where the harvestable biomass is greatest or where fishes are most accessible, or both. Fishing mortality is therefore selective with respect both to species and to phenotypic variation within species.[16][17][2] Moreover, even a uniform change in overall mortality is evolutionarily selective. This happens because mortality discounts fitness gains incurred in late life; traits such as maturation that involve trade-offs between fitness gains in early and late life are therefore affected by a change in mortality, selective or not.
There are strong indications that the observed changes have partly a genetic basis. However, it is difficult to distinguish genetic evolution from phenotypic plasticity, i.e., a tendency of phenotypic traits to take different values depending on the current environmental conditions. Rijnsdorp (1993)[18] carried out a study to disentangle the causes of a major phenotypic change in maturation of North Sea plaice (Pleuronectes platessa). He concluded that a substantial part of the change in maturation is consistent with genetic change caused by fishing. Simply through the action of fishing, fisheries generate selection, causing evolution in life-history traits. More recently, similar results have been obtained for many different fish populations[19] [20], although the extent to which the documented changes are genetic versus environmental remains debated[21].
The common trend is a decreased age-at-maturation in heavily exploited fish stocks[19] [20], but this selection pattern is not always consistent. For instance, there are two spatially separated fisheries targeting cod (Gadus morhua) in the Barents Sea: a feeder (exploitation of the stock on the feeding grounds) and a spawner fishery (exploitation of the stock on the spawning grounds). Fishing confined to the spawning grounds gives an advantage to late maturation. This is because the extra mortality due to fishing on the spawning grounds makes it advantageous to grow for longer before maturation and thereby to produce more eggs when risking a visit to the spawning ground. If fishing mortality on the feeding grounds is added on, the relatively small advantage to late maturation is changed to a large advantage to early maturation.[22][23] Remaining on the feeding grounds is now itself risky, and a fish that does not mature until about, say 8 years of age is most likely to be caught before it spawns.
Current patterns of fishing generate strong selection for early maturation and, given appropriate genetic variation, substantial genetic change can be expected. But, if one were to try to reverse the process by closing the fishery, selection for later maturation would be weak. In other words, it could be hard to undo the effects of inadvertent selection caused by fishing.
Impact of discard
Indirect effects of physical disturbance
The resuspension, transport and subsequent deposition of sediment may affect the settlement and feeding of the biota in other areas.[2]
The potential effects of sediment resuspension include clogging of feeding apparatus or reduction of light availability[24] and sediment deposition has been shown to inhibit the settlement and growth of oysters and scallops.[25][26]
Mobile gears effects the epifauna by modification of substrata and removal of biogenic concretions and a consequent decline in the abundance of fauna associated with them. The loss of biogenic species not only reduces the supply of important prey species, but also increases predation risk for juvenile commercial species thereby lowering subsequent recruitment to the adult stocks.[27]
See also
Ecosystem effects of fishing in Mediterranean watersMarBEF examples of fishery effects
References
- ↑ Pauly, D. & Christensen, V.(1995). Primary production required to sustain global fisheries. Nature 374: 255-257.
- ↑ 2.0 2.1 2.2 2.3 2.4 2.5 2.6 2.7 Jennings, S.& Kaiser, M. (1998). The effects of fishing on marine ecosystems. Adv. Mar. Biol. 34: 201-352. Cite error: Invalid
<ref>
tag; name "Jennings1998" defined multiple times with different content Cite error: Invalid<ref>
tag; name "Jennings1998" defined multiple times with different content - ↑ Watson, R. & Pauly, D. (2001). Systematic distortion in world fisheries catch trends. Nature 414 (6863): 534-536.
- ↑ 4.0 4.1 Christensen, V.; Guénette, S.; Heymans, J.J. et al. (2003). Hundred-year decline of North Atlantic predatory fishes. Fish Fisher 4: 1-24. cit. in: Trites, A.W.; Christensen, V.; Pauly, D. (2006). Effects of fisheries on ecosystems: just another top predator?, in: Boyd, I.L. et al. (Ed.) (2006). Top predators in marine ecosystems: their role in monitoring and management. Conservation Biology 12:11-27. Cite error: Invalid
<ref>
tag; name "" defined multiple times with different content - ↑ Pauly, D.; Christenen, V.; Dalsgaard, J.; Froese, R.; Torres, F. Jr. (1998). Fishing Down Marine Food Webs. Science 279: 860-863.
- ↑ Morato, T.; Watson, R.; Pitcher, T., J. & Pauly, D. (2006). Fishing down the deep. fish and fisheries 7: 24-34.
- ↑ EEA report (2002). Europe’s biodiversity – biogeographical regions and seas – biogeographical regions in Europe – The Arctic Ocean
- ↑ Atkinson, R.J.A. & Nash, R.D.M. (1990). Some preliminary observations on the burrows of Callianassa subterranean (Montagu) (Decapoda: thalassinidae) from the west coast of Scotland. Journal of Natural History 24: 403-413. cit in: Jennings, S. & Kaiser, M. (1998). The effects of fishing on marine ecosystems. Adv. Mar. Biol. 34: 201-352.
- ↑ Riesen, W. & Riese, K. (1982). Macrobenthos of the subtidal Wadden Sea: reveisited after 55 years. Helgolander Meeresuntersuchungen 35: 409-423. cit in: Jennings, S.; Kaiser, M. (1998). The effects of fishing on marine ecosystems. Adv. Mar. Biol. 34: 201-352.
- ↑ Lindley, J.A.; Gamble, J.C. & Hunt, H.G. (1995). A change in the zooplankton of the central North Sea (55° to 58°N): a possible consequence of changes in the benthos. Marine Ecology Progress Series 119: 299-303. cit in: Jennings, S.; Kaiser, M. (1998). The effects of fishing on marine ecosystems. Adv. Mar. Biol. 34: 201-352.
- ↑ Miller, R.J. (1977). Resource underutilization in a spider crab industry. Fisheries 2: 9-13.
- ↑ Potter, E.C.E. & Pawson, M.G. (1991). Gill netting. Laboratory leaflets, MAFF, Directorate of Fisheries Research, Lowestoft 69, 34pp.
- ↑ Carr, H.A.; Blott, A.J. & Caruso, P.G. (1992). A study of ghost gillnets in the inshore waters of southern New England. In “MTS” 92: Global Ocean Partnership”, pp. 361-367. Marine Technology Society, Washington D.C. cit. in: Jennings, S.; Kaiser, M. (1998). The effects of fishing on marine ecosystems. Adv. Mar. Biol. 34: 201-352.
- ↑ Daskalov, G.M. (2002). Overfishing drives a trophic cascade in the Black Sea. Marine Ecology Progress Series 225: 53-63.
- ↑ Trites, A.; Christensen, V. & Pauly, D. (1997). Competition between fisheries and marine mammals for prey and primary production in the Pacific Ocean. Journal North West Atlantic Fisheries Science 22: 173-187. cit. in: Trites, A.W.; Christensen, V.; Pauly, D. (2006). Effects of fisheries on ecosystems: just another top predator?, in: Boyd, I.L. et al. (Ed.) (2006). Top predators in marine ecosystems: their role in monitoring and management. Conservation Biology 12: 11-27.
- ↑ Stokes, T.K.; McGlade, J.M. & Law, R. (eds) (1993). The exploitation of Evolving Resources. Lecture Notes in Biomathematics, 99. Springer-Verlag, Berlin. 264 pp.
- ↑ Law, R. (2000). Fishing, selection, and phenotypic evolution. ICES Journal of Marine Science 57: 659-668.
- ↑ Rijnsdorp, A.D. (1993). Fisheries as a large-scale experiment on life-history evolution: disentangling phenotypic and genetic effects in changes in maturation and reproduction of North Sea plaice, Pleuronectes platessa L. Oecologia 96:391-401.
- ↑ 19.0 19.1 Jørgensen, C., K. Enberg, E. S. Dunlop, et al. (2007) Managing evolving fish stocks. Science 318: 1247-1248.
- ↑ 20.0 20.1 Heino, M. and U. Dieckmann (2008) Detecting fisheries-induced life-history evolution: an overview of the reaction-norm approach. Bulletin of Marine Science 83: 69-93.
- ↑ Marshall, C. T. and H. I. Browman, eds. (2007) Disentangling the causes of maturation trends in exploited fish populations. Marine Ecology-Progress Series 335: 249–310.
- ↑ Borisov, V.M. (1978). The selective effect of fishing on the population structure of species with a long life cycle. Journal of Ichthyology 18: 896-904.
- ↑ Law, R. & Grey, D.R. (1989). Evolution of yields from populations with age-specific cropping. Evolutionary Ecology 3: 343-359.
- ↑ Rhoads, D.C. (1974). Organism-sediment relations on the muddy sea floor. Oceanography and Marine Biology Annual Review 12: 263-300. cit. in: Jennings, S. & Kaiser, M. (1998). The effects of fishing on marine ecosystems. Adv. Mar. Biol. 34: 201-352.
- ↑ Moore, P.G (1977). Inorganic particulate suspensions in the sea and their effects on marine animals. Oceanography and Marine Biology Annual Review 15: 225-363. cit. in: Jennings, S. & Kaiser, M. (1998). The effects of fishing on marine ecosystems. Adv. Mar. Biol. 34: 201-352.
- ↑ Jones, J.B. (1992). Environmental impact of trawling on the seabed: a review. New Zealand Journal of Marine and Freshwater research 26: 59-67. cit. in: Jennings, S.& Kaiser, M. (1998). The effects of fishing on marine ecosystems. Adv. Mar. Biol. 34: 201-352.
- ↑ Walters, C.J. & Juanes, F. (1993). Recruitment limitations as a consequence of natural selection for use of restricted feeding habitats and predation risk taking by juvenile fishes. Canadian Journal of Fisheries and Aquatic Science 50: 2058-2070. cit. in: Jennings, S.; Kaiser, M. (1998). The effects of fishing on marine ecosystems. Adv. Mar. Biol. 34: 201-352.
Please note that others may also have edited the contents of this article.
|